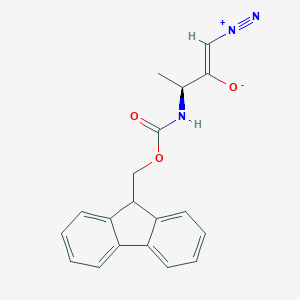
Fmoc-L-Alanin-CHN2
- Klicken Sie auf QUICK INQUIRY, um ein Angebot von unserem Expertenteam zu erhalten.
- Mit qualitativ hochwertigen Produkten zu einem WETTBEWERBSFÄHIGEN Preis können Sie sich mehr auf Ihre Forschung konzentrieren.
Übersicht
Beschreibung
Fmoc-L-Ala-CHN2 is a synthetic compound widely used in organic chemistry, particularly in peptide synthesis. The compound features a 9-fluorenylmethoxycarbonyl (Fmoc) group, which is a common protecting group for amines in peptide synthesis. The diazo group in the compound is known for its reactivity, making it a versatile intermediate in various chemical reactions.
Wissenschaftliche Forschungsanwendungen
Fmoc-L-Ala-CHN2 has several applications in scientific research:
Chemistry: Used in peptide synthesis as a protecting group for amines, facilitating the formation of peptide bonds.
Biology: Employed in the synthesis of biologically active peptides and proteins for research purposes.
Medicine: Utilized in the development of peptide-based drugs and therapeutic agents.
Industry: Applied in the production of specialty chemicals and pharmaceuticals.
Wirkmechanismus
Target of Action
Fmoc-L-Ala-CHN2, also known as N-alpha-(9-Fluorenylxycarbonyl)-L-alaninyl-diazomethane, is primarily used in proteomics studies and solid phase peptide synthesis techniques . Its primary targets are the amino acids in peptides that are being synthesized .
Mode of Action
Fmoc-L-Ala-CHN2 acts as a protecting group for the amino acid alanine during peptide synthesis . The Fmoc group is typically removed with a base such as pyridine . This is an orthogonal de-protection strategy to the acid labile Boc group .
Biochemical Pathways
The compound plays a crucial role in the chemical synthesis of peptides . It is involved in the formation of peptide bonds, which requires the activation of the carboxyl group of an amino acid and the protection of the Na-amino group . The Fmoc group allows for very rapid and highly efficient synthesis of peptides, including ones of significant size and complexity .
Pharmacokinetics
Its stability under certain conditions, such as treatment with trifluoroacetic acid (tfa) and hydrogen bromide/acetic acid, has been noted .
Result of Action
The use of Fmoc-L-Ala-CHN2 in peptide synthesis results in the formation of peptides with protected amino groups . These peptides can then be further modified or used in various applications, such as proteomics studies .
Action Environment
The action of Fmoc-L-Ala-CHN2 is influenced by the chemical environment. For instance, the Fmoc group is stable under acidic conditions but can be removed under basic conditions . Therefore, the pH of the environment can significantly influence the efficacy of Fmoc-L-Ala-CHN2 in peptide synthesis .
Biochemische Analyse
Biochemical Properties
Fmoc-L-Ala-CHN2 is potentially useful for proteomics studies and solid phase peptide synthesis techniques . The Fmoc group is typically removed with a base such as pyridine . This is an orthogonal de-protection strategy to the acid labile Boc group .
Molecular Mechanism
The molecular mechanism of Fmoc-L-Ala-CHN2 involves the protection of amine groups. The Fmoc group can be introduced by reacting the amine with fluorenylmethyloxycarbonyl chloride (Fmoc-Cl) . This mechanism is frequently used as a protecting group for amines .
Temporal Effects in Laboratory Settings
In laboratory settings, Fmoc-K(Fmoc) has shown to exhibit pH-controlled ambidextrous gelation, pH stimulus response, high thermal stability (∼100 °C) even at low minimum hydrogelation concentration (0.1 wt%), thixotropic property, high kinetic and mechanical stability, dye removal properties, cell viability to the selected cell type, and as a drug carrier .
Vorbereitungsmethoden
Synthetic Routes and Reaction Conditions
The synthesis of Fmoc-L-Ala-CHN2 typically involves the following steps:
Fmoc Protection: The Fmoc group is introduced to the amino acid using 9-fluorenylmethyloxycarbonyl chloride (Fmoc-Cl) in the presence of a base such as pyridine or triethylamine.
Diazomethane Formation: The diazo group is introduced by treating the Fmoc-protected amino acid with diazomethane or its derivatives under controlled conditions.
Industrial Production Methods
Industrial production of this compound follows similar synthetic routes but on a larger scale. The process involves:
Bulk Synthesis: Large quantities of Fmoc-Cl and diazomethane are used to produce the compound in bulk.
Purification: The product is purified using techniques such as crystallization or chromatography to ensure high purity and yield.
Analyse Chemischer Reaktionen
Types of Reactions
Fmoc-L-Ala-CHN2 undergoes various chemical reactions, including:
Oxidation: The diazo group can be oxidized to form ketones or carboxylic acids.
Reduction: The compound can be reduced to form amines or alcohols.
Substitution: The diazo group can be substituted with nucleophiles to form different derivatives.
Common Reagents and Conditions
Oxidation: Reagents such as potassium permanganate or chromium trioxide are used under acidic or basic conditions.
Reduction: Reagents like lithium aluminum hydride or sodium borohydride are used under mild conditions.
Substitution: Nucleophiles such as amines, alcohols, or thiols are used under basic conditions.
Major Products Formed
Oxidation: Ketones, carboxylic acids.
Reduction: Amines, alcohols.
Substitution: Various substituted derivatives depending on the nucleophile used.
Vergleich Mit ähnlichen Verbindungen
Similar Compounds
- N-alpha-(9-Fluorenylxycarbonyl)-3-(3-pyridyl)-L-alanine
- N-alpha-t-butyloxycarbonyl-N-epsilon-(9-fluorenylmethyloxycarbonyl)-D-lysine
Uniqueness
Fmoc-L-Ala-CHN2 is unique due to its combination of the Fmoc protecting group and the diazo group. This dual functionality allows for versatile applications in peptide synthesis and other chemical transformations, making it a valuable compound in both research and industrial settings.
Eigenschaften
IUPAC Name |
9H-fluoren-9-ylmethyl N-[(2S)-4-diazo-3-oxobutan-2-yl]carbamate |
Source
|
---|---|---|
Source | PubChem | |
URL | https://pubchem.ncbi.nlm.nih.gov | |
Description | Data deposited in or computed by PubChem | |
InChI |
InChI=1S/C19H17N3O3/c1-12(18(23)10-21-20)22-19(24)25-11-17-15-8-4-2-6-13(15)14-7-3-5-9-16(14)17/h2-10,12,17H,11H2,1H3,(H,22,24)/t12-/m0/s1 |
Source
|
Source | PubChem | |
URL | https://pubchem.ncbi.nlm.nih.gov | |
Description | Data deposited in or computed by PubChem | |
InChI Key |
MGOXETIYWQKLEA-LBPRGKRZSA-N |
Source
|
Source | PubChem | |
URL | https://pubchem.ncbi.nlm.nih.gov | |
Description | Data deposited in or computed by PubChem | |
Canonical SMILES |
CC(C(=O)C=[N+]=[N-])NC(=O)OCC1C2=CC=CC=C2C3=CC=CC=C13 |
Source
|
Source | PubChem | |
URL | https://pubchem.ncbi.nlm.nih.gov | |
Description | Data deposited in or computed by PubChem | |
Isomeric SMILES |
C[C@@H](C(=O)C=[N+]=[N-])NC(=O)OCC1C2=CC=CC=C2C3=CC=CC=C13 |
Source
|
Source | PubChem | |
URL | https://pubchem.ncbi.nlm.nih.gov | |
Description | Data deposited in or computed by PubChem | |
Molecular Formula |
C19H17N3O3 |
Source
|
Source | PubChem | |
URL | https://pubchem.ncbi.nlm.nih.gov | |
Description | Data deposited in or computed by PubChem | |
Molecular Weight |
335.4 g/mol |
Source
|
Source | PubChem | |
URL | https://pubchem.ncbi.nlm.nih.gov | |
Description | Data deposited in or computed by PubChem | |
Haftungsausschluss und Informationen zu In-Vitro-Forschungsprodukten
Bitte beachten Sie, dass alle Artikel und Produktinformationen, die auf BenchChem präsentiert werden, ausschließlich zu Informationszwecken bestimmt sind. Die auf BenchChem zum Kauf angebotenen Produkte sind speziell für In-vitro-Studien konzipiert, die außerhalb lebender Organismen durchgeführt werden. In-vitro-Studien, abgeleitet von dem lateinischen Begriff "in Glas", beinhalten Experimente, die in kontrollierten Laborumgebungen unter Verwendung von Zellen oder Geweben durchgeführt werden. Es ist wichtig zu beachten, dass diese Produkte nicht als Arzneimittel oder Medikamente eingestuft sind und keine Zulassung der FDA für die Vorbeugung, Behandlung oder Heilung von medizinischen Zuständen, Beschwerden oder Krankheiten erhalten haben. Wir müssen betonen, dass jede Form der körperlichen Einführung dieser Produkte in Menschen oder Tiere gesetzlich strikt untersagt ist. Es ist unerlässlich, sich an diese Richtlinien zu halten, um die Einhaltung rechtlicher und ethischer Standards in Forschung und Experiment zu gewährleisten.