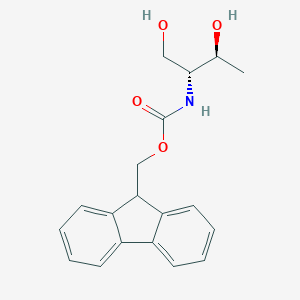
Fmoc-L-allo-threoninol
Übersicht
Beschreibung
Fmoc-L-allo-threoninol: is a derivative of threonine, an amino acid, modified with a fluorenylmethyloxycarbonyl (Fmoc) protecting group. This compound is commonly used in peptide synthesis due to its ability to protect the amine group during chemical reactions. The Fmoc group is base-labile, meaning it can be removed under basic conditions, making it a versatile tool in organic synthesis .
Vorbereitungsmethoden
Synthetic Routes and Reaction Conditions: Fmoc-L-allo-threoninol can be synthesized through the reaction of N-(9-fluorenylmethoxycarbonyloxy)succinimide with d-threoninol . The reaction typically involves the use of a base such as sodium bicarbonate in an aqueous dioxane solution to facilitate the formation of the Fmoc-protected compound .
Industrial Production Methods: Industrial production of this compound follows similar synthetic routes but on a larger scale. The process involves the use of automated peptide synthesizers and large-scale reactors to ensure high yield and purity. The reaction conditions are optimized to minimize side reactions and maximize the efficiency of the Fmoc protection .
Analyse Chemischer Reaktionen
Types of Reactions: Fmoc-L-allo-threoninol undergoes various chemical reactions, including:
Oxidation: The hydroxyl group in threoninol can be oxidized to form a ketone or aldehyde.
Reduction: The compound can be reduced to form a primary alcohol.
Substitution: The hydroxyl group can be substituted with other functional groups through nucleophilic substitution reactions.
Common Reagents and Conditions:
Oxidation: Reagents such as pyridinium chlorochromate (PCC) or Dess-Martin periodinane are commonly used.
Reduction: Sodium borohydride (NaBH4) or lithium aluminum hydride (LiAlH4) are typical reducing agents.
Substitution: Nucleophiles such as halides or amines can be used under basic conditions.
Major Products:
Oxidation: Formation of ketones or aldehydes.
Reduction: Formation of primary alcohols.
Substitution: Formation of substituted threoninol derivatives.
Wissenschaftliche Forschungsanwendungen
Chemistry: Fmoc-L-allo-threoninol is widely used in peptide synthesis as a protecting group for the amine functionality. It allows for the sequential addition of amino acids to form peptides and proteins .
Biology: In biological research, this compound is used to study protein-protein interactions and enzyme-substrate interactions. It is also used in the synthesis of peptide-based drugs and biomaterials .
Medicine: The compound is utilized in the development of peptide-based therapeutics, including antibiotics and anticancer agents. Its ability to protect the amine group during synthesis makes it valuable in the production of complex peptide drugs .
Industry: this compound is used in the production of functional materials, such as hydrogels and nanomaterials, due to its self-assembly properties. It is also employed in the synthesis of catalysts and other industrial chemicals .
Wirkmechanismus
The mechanism of action of Fmoc-L-allo-threoninol involves the protection of the amine group through the formation of a stable carbamate linkage with the Fmoc group. This protects the amine from unwanted reactions during peptide synthesis. The Fmoc group can be removed under basic conditions, typically using piperidine, to reveal the free amine group for further reactions .
Vergleich Mit ähnlichen Verbindungen
Fmoc-L-threoninol: Similar to Fmoc-L-allo-threoninol but with a different stereochemistry.
Fmoc-L-serinol: Another Fmoc-protected amino alcohol used in peptide synthesis.
Fmoc-L-tyrosinol: Contains an aromatic ring in addition to the Fmoc group and hydroxyl functionality.
Uniqueness: this compound is unique due to its specific stereochemistry, which can influence the properties and reactivity of the resulting peptides. Its ability to form stable carbamate linkages and its ease of removal under basic conditions make it a valuable tool in peptide synthesis .
Biologische Aktivität
Fmoc-L-allo-threoninol, a derivative of threonine, has garnered attention in biochemical research due to its potential biological activities. This article explores its synthesis, biological properties, and applications, drawing from diverse sources to provide a comprehensive overview.
This compound is characterized by the following chemical properties:
- Molecular Formula : C19H19NO5
- Molecular Weight : 341.36 g/mol
- Density : 1.3 g/cm³
- Boiling Point : 596.5 °C at 760 mmHg
This compound is often used in peptide synthesis due to its protective Fmoc (9-fluorenylmethoxycarbonyl) group, which allows for selective reactions without affecting other functional groups.
Synthesis
The synthesis of this compound typically involves:
- Protection of the hydroxyl groups using the Fmoc group.
- Coupling with other amino acids or derivatives through standard peptide coupling techniques.
- Deprotection to yield the final product.
The methods employed can vary, but common strategies include the use of coupling reagents like DIC (N,N'-diisopropylcarbodiimide) and DMAP (4-dimethylaminopyridine) to facilitate the formation of peptide bonds.
Anticancer Activity
Recent studies have evaluated the anticancer properties of various threonine derivatives, including this compound. For instance, a study assessing the cytotoxic effects on Huh-7 cancer cells demonstrated that certain synthetic peptides derived from threonine exhibited low cytotoxicity (IC50 > 50 μM), indicating limited anticancer activity compared to established chemotherapeutics like 5-fluorouracil (IC50 = 15.3 μM) .
Table 1: Biological Activity Summary of Threonine Derivatives
Compound | Activity Type | IC50 (μM) | Reference |
---|---|---|---|
This compound | Anticancer | >50 | |
5-Fluorouracil | Anticancer | 15.3 | |
Compound from Zelkovamycin family | Antibacterial | 0.62 - 2.5 |
The mechanism by which this compound exerts its biological effects is not fully elucidated. However, it is hypothesized that its interaction with cellular pathways may involve modulation of protein synthesis and cellular signaling cascades relevant to cancer progression and microbial resistance.
Eigenschaften
IUPAC Name |
9H-fluoren-9-ylmethyl N-[(2R,3S)-1,3-dihydroxybutan-2-yl]carbamate | |
---|---|---|
Source | PubChem | |
URL | https://pubchem.ncbi.nlm.nih.gov | |
Description | Data deposited in or computed by PubChem | |
InChI |
InChI=1S/C19H21NO4/c1-12(22)18(10-21)20-19(23)24-11-17-15-8-4-2-6-13(15)14-7-3-5-9-16(14)17/h2-9,12,17-18,21-22H,10-11H2,1H3,(H,20,23)/t12-,18+/m0/s1 | |
Source | PubChem | |
URL | https://pubchem.ncbi.nlm.nih.gov | |
Description | Data deposited in or computed by PubChem | |
InChI Key |
YOKDHMTZJSRRIQ-KPZWWZAWSA-N | |
Source | PubChem | |
URL | https://pubchem.ncbi.nlm.nih.gov | |
Description | Data deposited in or computed by PubChem | |
Canonical SMILES |
CC(C(CO)NC(=O)OCC1C2=CC=CC=C2C3=CC=CC=C13)O | |
Source | PubChem | |
URL | https://pubchem.ncbi.nlm.nih.gov | |
Description | Data deposited in or computed by PubChem | |
Isomeric SMILES |
C[C@@H]([C@@H](CO)NC(=O)OCC1C2=CC=CC=C2C3=CC=CC=C13)O | |
Source | PubChem | |
URL | https://pubchem.ncbi.nlm.nih.gov | |
Description | Data deposited in or computed by PubChem | |
Molecular Formula |
C19H21NO4 | |
Source | PubChem | |
URL | https://pubchem.ncbi.nlm.nih.gov | |
Description | Data deposited in or computed by PubChem | |
DSSTOX Substance ID |
DTXSID00426524 | |
Record name | (9H-Fluoren-9-yl)methyl [(2R,3S)-1,3-dihydroxybutan-2-yl]carbamate | |
Source | EPA DSSTox | |
URL | https://comptox.epa.gov/dashboard/DTXSID00426524 | |
Description | DSSTox provides a high quality public chemistry resource for supporting improved predictive toxicology. | |
Molecular Weight |
327.4 g/mol | |
Source | PubChem | |
URL | https://pubchem.ncbi.nlm.nih.gov | |
Description | Data deposited in or computed by PubChem | |
CAS No. |
252049-03-9 | |
Record name | (9H-Fluoren-9-yl)methyl [(2R,3S)-1,3-dihydroxybutan-2-yl]carbamate | |
Source | EPA DSSTox | |
URL | https://comptox.epa.gov/dashboard/DTXSID00426524 | |
Description | DSSTox provides a high quality public chemistry resource for supporting improved predictive toxicology. | |
Haftungsausschluss und Informationen zu In-Vitro-Forschungsprodukten
Bitte beachten Sie, dass alle Artikel und Produktinformationen, die auf BenchChem präsentiert werden, ausschließlich zu Informationszwecken bestimmt sind. Die auf BenchChem zum Kauf angebotenen Produkte sind speziell für In-vitro-Studien konzipiert, die außerhalb lebender Organismen durchgeführt werden. In-vitro-Studien, abgeleitet von dem lateinischen Begriff "in Glas", beinhalten Experimente, die in kontrollierten Laborumgebungen unter Verwendung von Zellen oder Geweben durchgeführt werden. Es ist wichtig zu beachten, dass diese Produkte nicht als Arzneimittel oder Medikamente eingestuft sind und keine Zulassung der FDA für die Vorbeugung, Behandlung oder Heilung von medizinischen Zuständen, Beschwerden oder Krankheiten erhalten haben. Wir müssen betonen, dass jede Form der körperlichen Einführung dieser Produkte in Menschen oder Tiere gesetzlich strikt untersagt ist. Es ist unerlässlich, sich an diese Richtlinien zu halten, um die Einhaltung rechtlicher und ethischer Standards in Forschung und Experiment zu gewährleisten.