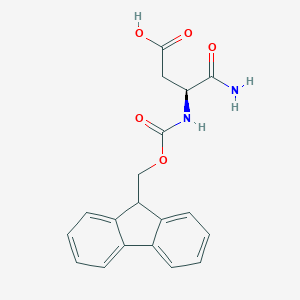
Fmoc-Asp-NH2
Übersicht
Beschreibung
Fmoc-Asp-NH2, also known as fluorenylmethyloxycarbonyl-L-asparagine, is a derivative of aspartic acid. It is commonly used in solid-phase peptide synthesis as a protecting group for the amino group. The fluorenylmethyloxycarbonyl group is base-labile, making it suitable for use in peptide synthesis where mild deprotection conditions are required .
Vorbereitungsmethoden
Synthetic Routes and Reaction Conditions: Fmoc-Asp-NH2 can be synthesized through the reaction of aspartic acid with fluorenylmethyloxycarbonyl chloride in the presence of a base such as sodium bicarbonate. The reaction typically takes place in an organic solvent like dioxane . Another method involves the use of 9-fluorenylmethylsuccinimidyl carbonate, which reacts with the dicyclohexylammonium salt of N-hydroxysuccinimide .
Industrial Production Methods: In industrial settings, the production of this compound follows similar synthetic routes but on a larger scale. The process involves the use of automated peptide synthesizers and high-purity reagents to ensure the consistency and quality of the final product .
Analyse Chemischer Reaktionen
Types of Reactions: Fmoc-Asp-NH2 undergoes several types of chemical reactions, including:
Deprotection: The fluorenylmethyloxycarbonyl group is removed using a base such as piperidine in N,N-dimethylformamide.
Coupling Reactions: It can participate in peptide bond formation through reactions with other amino acids or peptides.
Common Reagents and Conditions:
Deprotection: Piperidine in N,N-dimethylformamide is commonly used for the removal of the fluorenylmethyloxycarbonyl group.
Major Products:
Deprotection: The major product is the free amine form of asparagine.
Coupling: The major products are peptides with the desired sequence.
Wissenschaftliche Forschungsanwendungen
Peptide Synthesis
Overview
Fmoc-Asp-NH2 is primarily used in solid-phase peptide synthesis (SPPS) due to its ability to form stable peptide bonds while minimizing side reactions. The Fmoc group serves as a protecting group for the amino group, allowing for selective coupling and deprotection steps during the synthesis process.
Case Study: Overcoming Aspartimide Formation
Research has shown that using this compound significantly reduces the formation of aspartimide, a common by-product in peptide synthesis. In a comparative study involving various aspartic acid derivatives, this compound demonstrated superior performance with negligible aspartimide formation even after prolonged exposure to deprotecting conditions (piperidine in DMF) . This property enhances the purity of the synthesized peptides, making this compound a preferred choice in complex peptide synthesis.
Biological Research
Protein-Protein Interactions
this compound is instrumental in studying protein-protein interactions. By incorporating this compound into peptide sequences, researchers can investigate binding affinities and interaction dynamics between proteins, aiding in understanding cellular signaling pathways and metabolic processes.
Enzyme-Substrate Interactions
The compound's role extends to enzyme-substrate interactions where it serves as a model substrate for characterizing enzyme activity. Its structural properties allow for the design of experiments that elucidate enzyme mechanisms and substrate specificity.
Medicinal Chemistry
Drug Development
In medicinal chemistry, this compound is utilized in the synthesis of peptide-based drugs and therapeutic agents. Its ability to form bioactive peptides makes it a valuable tool in drug discovery. For instance, researchers have developed antibody-drug conjugates (ADCs) using this compound as a linker, enhancing the targeted delivery of therapeutic agents to cancer cells .
Vaccine Development
The compound also finds applications in vaccine development by facilitating the synthesis of peptide antigens that can elicit immune responses. This application is particularly relevant in designing vaccines against infectious diseases and cancer.
Industrial Applications
Peptide-based Materials
this compound is employed in producing peptide-based materials such as hydrogels and nanomaterials. These materials have significant implications in tissue engineering and regenerative medicine due to their biocompatibility and ability to mimic natural extracellular matrices .
Case Study: Hydrogels for Tissue Engineering
Recent studies have demonstrated the formation of self-assembling hydrogels from Fmoc-Asp derivatives. These hydrogels exhibit excellent mechanical properties and can support cell growth and differentiation, making them suitable candidates for applications in tissue repair and regeneration .
Summary Table of Applications
Field | Application | Details |
---|---|---|
Chemistry | Peptide Synthesis | Used as a building block in SPPS; minimizes aspartimide formation |
Biology | Protein-Protein Interactions | Investigates binding affinities; aids understanding of signaling pathways |
Enzyme-Substrate Interactions | Serves as a model substrate for characterizing enzyme activity | |
Medicine | Drug Development | Utilized in synthesizing peptide-based drugs; ADC linkers for targeted therapy |
Vaccine Development | Facilitates synthesis of peptide antigens for immune response | |
Industry | Peptide-based Materials | Used in producing hydrogels for tissue engineering; mimics extracellular matrices |
Wirkmechanismus
The mechanism of action of Fmoc-Asp-NH2 involves the protection of the amino group of asparagine, preventing unwanted side reactions during peptide synthesis. The fluorenylmethyloxycarbonyl group is removed under basic conditions, allowing the free amine to participate in subsequent coupling reactions . The molecular targets and pathways involved include the formation of peptide bonds and the stabilization of intermediate structures during synthesis .
Vergleich Mit ähnlichen Verbindungen
Fmoc-Gly-NH2 (fluorenylmethyloxycarbonyl-glycine): Similar in function but used for glycine residues.
Fmoc-Lys-NH2 (fluorenylmethyloxycarbonyl-lysine): Used for lysine residues and has a similar deprotection mechanism.
Uniqueness: Fmoc-Asp-NH2 is unique due to its specific application in the synthesis of peptides containing asparagine. Its ability to form stable intermediates and its compatibility with mild deprotection conditions make it a valuable tool in peptide synthesis .
Biologische Aktivität
Fmoc-Asp-NH2 (Fluorenylmethyloxycarbonyl-L-aspartic acid amide) is a derivative of aspartic acid that plays a significant role in peptide synthesis and has various biological applications. This article provides a detailed overview of its biological activity, including its synthesis, mechanisms of action, and implications in therapeutic contexts.
Overview of this compound
This compound is primarily utilized in solid-phase peptide synthesis (SPPS) due to its stability and ease of handling. The Fmoc group protects the amino group during synthesis, allowing for selective deprotection and subsequent coupling with other amino acids. Its unique properties make it a valuable building block in the development of peptides with specific biological functions.
Antimicrobial Properties
This compound has been investigated for its antimicrobial properties. Studies have shown that analogs of antimicrobial peptides containing aspartic acid residues exhibit varying degrees of activity against different bacterial strains. For instance, modifications to the Fmoc-Asp structure can enhance its efficacy against Gram-positive bacteria like Staphylococcus aureus and Gram-negative bacteria such as Escherichia coli .
Table 1: Antimicrobial Activity of Fmoc-Asp Derivatives
Compound | MIC (μM) against E. coli | MIC (μM) against S. aureus |
---|---|---|
Native this compound | 2 | 4 |
Lactam-bridged analog | 4 | 8 |
Bicyclic analogue | 16 | 32 |
These findings suggest that structural modifications to this compound can significantly influence its biological activity, particularly its antimicrobial efficacy.
Peptide Synthesis and Aspartimide Formation
One notable challenge in the synthesis of peptides containing aspartic acid is the formation of aspartimide, a side reaction that can lead to chain termination during SPPS. Research indicates that the choice of protecting groups and deprotection conditions can mitigate this issue. For example, using Fmoc-Asp(OBno)-OH has been shown to reduce aspartimide formation significantly, resulting in higher yields and purities of target peptides (see Table 2).
Table 2: Comparison of Aspartimide Formation with Different Protecting Groups
Protecting Group | Aspartimide Formation (%) |
---|---|
Fmoc-Asp(OtBu)-OH | 15 |
Fmoc-Asp(OMpe)-OH | 10 |
Fmoc-Asp(OBno)-OH | 0.1 |
Case Study 1: Antibody-Drug Conjugates (ADCs)
This compound serves as a cleavable linker in the synthesis of antibody-drug conjugates (ADCs). ADCs leverage the targeting ability of antibodies to deliver cytotoxic drugs selectively to cancer cells. The incorporation of this compound allows for controlled release mechanisms, enhancing therapeutic efficacy while minimizing off-target effects .
Case Study 2: Hydrogel Applications
Recent research has explored the use of Fmoc-Asp-based hydrogels for cell culture applications. These hydrogels provide a supportive environment for cell growth and differentiation, demonstrating potential in tissue engineering and regenerative medicine . The amphiphilic nature of these peptides facilitates self-assembly into structures that mimic extracellular matrices.
The biological activity of this compound is largely attributed to its ability to interact with cellular membranes and modulate membrane permeability. The cation-dependent activity profile observed in various studies suggests that these peptides may disrupt bacterial membranes, leading to cell lysis . Furthermore, the conformational flexibility afforded by the Fmoc group allows for diverse interactions with target molecules, enhancing their functional versatility.
Q & A
Basic Research Questions
Q. What are the key synthetic routes for preparing Fmoc-Asp-NH2, and how do side-chain protection strategies influence purity?
this compound synthesis typically involves Fmoc-group protection of the α-amino group, followed by selective activation of the β-carboxylic acid for amidation. Side-chain protection (e.g., using tert-butyl or benzyl esters) is critical to avoid undesired coupling during solid-phase peptide synthesis (SPPS). For example, Fmoc-Asp(OMpe)-OH and Fmoc-Asp(OBzl)-OH derivatives are used to block the β-carboxyl group, ensuring regioselective amidation . Post-synthesis, deprotection under mild acidic conditions (e.g., TFA) yields the final product. Purity is assessed via reverse-phase HPLC (>95%) and NMR to confirm the absence of residual protecting groups.
Q. Which analytical techniques are most reliable for characterizing this compound and its intermediates?
Structural confirmation requires tandem techniques:
- NMR : H and C NMR verify backbone connectivity and side-chain deprotection. For example, the Fmoc group’s aromatic protons appear at 7.3–7.8 ppm .
- HPLC : Reverse-phase HPLC with UV detection (254 nm) assesses purity, with retention times calibrated against known standards.
- Mass Spectrometry : High-resolution MS (e.g., ESI-TOF) confirms molecular weight (Mw = 354.35 g/mol for this compound) and detects impurities . Consistency in data reporting (e.g., ±0.1% mass accuracy) is essential for reproducibility .
Q. How does this compound function as a cleavable linker in antibody-drug conjugates (ADCs)?
The β-amide bond in this compound is susceptible to enzymatic cleavage (e.g., by cathepsin B in lysosomal environments), enabling controlled drug release in ADCs. This cleavage mechanism is pH-dependent, with optimal activity at pH 4.5–5.5 . Researchers must validate cleavage efficiency using:
- In vitro assays : Incubate ADCs with lysosomal enzymes and quantify drug release via LC-MS.
- Cell-based models : Measure cytotoxicity in target vs. non-target cells to confirm linker specificity .
Advanced Research Questions
Q. What experimental strategies optimize this compound’s stability during ADC storage and circulation?
Stability challenges include hydrolysis in plasma and aggregation. Mitigation strategies:
- Formulation buffers : Use lyophilization with trehalose (5% w/v) to prevent hydrolysis.
- Conjugation site : Attach the linker to cysteine residues (vs. lysine) for improved serum stability .
- Accelerated stability studies : Store ADCs at 40°C/75% RH for 4 weeks and monitor degradation via SEC-HPLC .
Q. How can researchers resolve contradictions in reported cleavage efficiencies of this compound-based ADCs?
Discrepancies often arise from variability in:
- Enzyme batches : Standardize cathepsin B activity (e.g., 0.1 U/µg ADC) across experiments.
- Drug-to-antibody ratio (DAR) : Optimize DAR (2–4) to balance efficacy and aggregation .
- Analytical methods : Use orthogonal techniques (e.g., fluorescence quenching and LC-MS) to cross-validate drug release .
Q. What computational tools predict this compound’s compatibility with novel payloads in ADC design?
Molecular dynamics (MD) simulations can model linker-payload interactions:
- Software : AMBER or GROMACS for energy minimization and binding affinity calculations.
- Parameters : Solubility (logP < 3) and steric hindrance between the linker and antibody . Validate predictions with SPR (surface plasmon resonance) to measure binding kinetics .
Q. How do modifications to the aspartic acid residue impact this compound’s performance in proteolysis-resistant peptides?
Substituting L-Asp with D-Asp (Fmoc-D-Asp-NH2) or isoasparagine (Fmoc-IsoAsn-OH) alters enzymatic cleavage rates:
- D-Asp : Reduces cathepsin B cleavage by 60% due to stereochemical incompatibility .
- Isoasparagine : Increases plasma stability but requires harsher deprotection conditions (e.g., 95% TFA) . See table in FAQ 1 for derivative properties.
Q. Methodological Guidelines
Q. What protocols ensure reproducible synthesis of this compound on a milligram-to-gram scale?
- Small scale (mg) : Use automated SPPS with HBTU/HOBt activation and DIPEA (2 eq) in DMF.
- Large scale (g) : Employ batch-wise coupling with pre-activated Fmoc-Asp-Opfp esters for higher yields . Document all steps in line with IUPAC nomenclature and journal-specific formatting (e.g., Beilstein J. Org. Chem. guidelines) .
Q. How should researchers present conflicting data on this compound’s cytotoxicity in peer-reviewed manuscripts?
- Transparency : Report raw data (mean ± SD) and statistical tests (e.g., ANOVA with Tukey post-hoc).
- Discussion : Address limitations (e.g., cell line variability) and propose follow-up experiments .
Q. What are the ethical considerations when designing in vivo studies with this compound-based ADCs?
Eigenschaften
IUPAC Name |
(3S)-4-amino-3-(9H-fluoren-9-ylmethoxycarbonylamino)-4-oxobutanoic acid | |
---|---|---|
Source | PubChem | |
URL | https://pubchem.ncbi.nlm.nih.gov | |
Description | Data deposited in or computed by PubChem | |
InChI |
InChI=1S/C19H18N2O5/c20-18(24)16(9-17(22)23)21-19(25)26-10-15-13-7-3-1-5-11(13)12-6-2-4-8-14(12)15/h1-8,15-16H,9-10H2,(H2,20,24)(H,21,25)(H,22,23)/t16-/m0/s1 | |
Source | PubChem | |
URL | https://pubchem.ncbi.nlm.nih.gov | |
Description | Data deposited in or computed by PubChem | |
InChI Key |
VHRMWRHTRSQVJJ-INIZCTEOSA-N | |
Source | PubChem | |
URL | https://pubchem.ncbi.nlm.nih.gov | |
Description | Data deposited in or computed by PubChem | |
Canonical SMILES |
C1=CC=C2C(=C1)C(C3=CC=CC=C32)COC(=O)NC(CC(=O)O)C(=O)N | |
Source | PubChem | |
URL | https://pubchem.ncbi.nlm.nih.gov | |
Description | Data deposited in or computed by PubChem | |
Isomeric SMILES |
C1=CC=C2C(=C1)C(C3=CC=CC=C32)COC(=O)N[C@@H](CC(=O)O)C(=O)N | |
Source | PubChem | |
URL | https://pubchem.ncbi.nlm.nih.gov | |
Description | Data deposited in or computed by PubChem | |
Molecular Formula |
C19H18N2O5 | |
Source | PubChem | |
URL | https://pubchem.ncbi.nlm.nih.gov | |
Description | Data deposited in or computed by PubChem | |
DSSTOX Substance ID |
DTXSID50426776 | |
Record name | Fmoc-Asp-NH2 | |
Source | EPA DSSTox | |
URL | https://comptox.epa.gov/dashboard/DTXSID50426776 | |
Description | DSSTox provides a high quality public chemistry resource for supporting improved predictive toxicology. | |
Molecular Weight |
354.4 g/mol | |
Source | PubChem | |
URL | https://pubchem.ncbi.nlm.nih.gov | |
Description | Data deposited in or computed by PubChem | |
CAS No. |
200335-40-6 | |
Record name | Fmoc-Asp-NH2 | |
Source | EPA DSSTox | |
URL | https://comptox.epa.gov/dashboard/DTXSID50426776 | |
Description | DSSTox provides a high quality public chemistry resource for supporting improved predictive toxicology. | |
Retrosynthesis Analysis
AI-Powered Synthesis Planning: Our tool employs the Template_relevance Pistachio, Template_relevance Bkms_metabolic, Template_relevance Pistachio_ringbreaker, Template_relevance Reaxys, Template_relevance Reaxys_biocatalysis model, leveraging a vast database of chemical reactions to predict feasible synthetic routes.
One-Step Synthesis Focus: Specifically designed for one-step synthesis, it provides concise and direct routes for your target compounds, streamlining the synthesis process.
Accurate Predictions: Utilizing the extensive PISTACHIO, BKMS_METABOLIC, PISTACHIO_RINGBREAKER, REAXYS, REAXYS_BIOCATALYSIS database, our tool offers high-accuracy predictions, reflecting the latest in chemical research and data.
Strategy Settings
Precursor scoring | Relevance Heuristic |
---|---|
Min. plausibility | 0.01 |
Model | Template_relevance |
Template Set | Pistachio/Bkms_metabolic/Pistachio_ringbreaker/Reaxys/Reaxys_biocatalysis |
Top-N result to add to graph | 6 |
Feasible Synthetic Routes
Haftungsausschluss und Informationen zu In-Vitro-Forschungsprodukten
Bitte beachten Sie, dass alle Artikel und Produktinformationen, die auf BenchChem präsentiert werden, ausschließlich zu Informationszwecken bestimmt sind. Die auf BenchChem zum Kauf angebotenen Produkte sind speziell für In-vitro-Studien konzipiert, die außerhalb lebender Organismen durchgeführt werden. In-vitro-Studien, abgeleitet von dem lateinischen Begriff "in Glas", beinhalten Experimente, die in kontrollierten Laborumgebungen unter Verwendung von Zellen oder Geweben durchgeführt werden. Es ist wichtig zu beachten, dass diese Produkte nicht als Arzneimittel oder Medikamente eingestuft sind und keine Zulassung der FDA für die Vorbeugung, Behandlung oder Heilung von medizinischen Zuständen, Beschwerden oder Krankheiten erhalten haben. Wir müssen betonen, dass jede Form der körperlichen Einführung dieser Produkte in Menschen oder Tiere gesetzlich strikt untersagt ist. Es ist unerlässlich, sich an diese Richtlinien zu halten, um die Einhaltung rechtlicher und ethischer Standards in Forschung und Experiment zu gewährleisten.