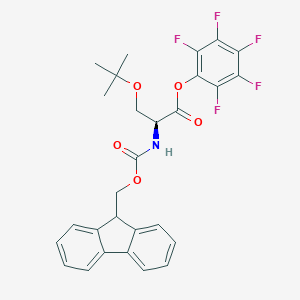
Fmoc-Ser(tBu)-OPfp
Übersicht
Beschreibung
Fmoc-Ser(tBu)-OPfp, also known as N-α-Fmoc-O-tert-butyl-L-serine pentafluorophenyl ester, is a derivative of serine used in peptide synthesis. It is a protected amino acid that facilitates the incorporation of serine residues into peptides during solid-phase peptide synthesis. The compound is characterized by the presence of a fluorenylmethyloxycarbonyl (Fmoc) group, a tert-butyl (tBu) group, and a pentafluorophenyl (Pfp) ester, which collectively enhance its stability and reactivity in peptide synthesis.
Wissenschaftliche Forschungsanwendungen
Festphasen-Peptidsynthese (SPPS)
Fmoc-Ser(tBu)-OPfp: wird häufig in der SPPS verwendet, einem Verfahren zur schrittweisen Synthese von Peptiden. Diese Verbindung mit ihrer schützenden Fmoc-Gruppe ermöglicht die sequenzielle Addition von Aminosäuren an eine wachsende Peptidkette, während gleichzeitig Nebenreaktionen minimiert werden .
Entwicklung von Peptidarzneimitteln
In der medizinischen Chemie spielt This compound eine entscheidende Rolle bei der Synthese von peptid-basierten Medikamenten. Seine Verwendung bei der Herstellung komplexer Peptide hat Auswirkungen auf die Entwicklung neuer Therapeutika, insbesondere im Bereich der Antibiotikasynthese, wie beispielsweise die Totalsynthese von Daptomycin .
Grüne Chemie
Die Verwendung von This compound steht im Einklang mit den Prinzipien der grünen Chemie in der Peptidsynthese. Es ermöglicht die Reduzierung des Einsatzes gefährlicher Lösungsmittel und der Abfallerzeugung und trägt so zu nachhaltigeren Laborpraktiken bei .
Wirkmechanismus
Target of Action
Fmoc-Ser(tBu)-OPfp is primarily used in the field of peptide synthesis . Its primary target is the peptide chain where it is incorporated as a serine residue . The role of this compound is to facilitate the addition of a serine residue to the peptide chain during the synthesis process .
Mode of Action
This compound interacts with its target by being incorporated into the peptide chain during the synthesis process . The Fmoc group serves as a protective group for the amino acid serine during the synthesis, preventing unwanted reactions . Once the serine residue has been successfully added to the peptide chain, the Fmoc group is removed, allowing the synthesis to proceed .
Biochemical Pathways
The incorporation of this compound into a peptide chain is part of the larger biochemical pathway of peptide synthesis . This process involves the sequential addition of amino acids to a growing peptide chain . The addition of a serine residue via this compound can influence the structure and function of the final peptide, as serine is often involved in enzyme active sites and protein-protein interactions .
Result of Action
The result of the action of this compound is the successful incorporation of a serine residue into a peptide chain . This can influence the biological activity of the final peptide product, as serine residues can participate in various biochemical interactions .
Action Environment
The action of this compound is typically carried out in a controlled laboratory environment during the process of solid-phase peptide synthesis . Factors such as temperature, solvent choice, and reaction time can influence the efficiency and success of the serine incorporation . Efforts have been made to use greener solvents in solid-phase peptide synthesis, which can reduce environmental impact without impairing the synthetic process .
Biochemische Analyse
Biochemical Properties
Fmoc-Ser(tBu)-OPfp plays a significant role in biochemical reactions, particularly in the synthesis of peptides . It interacts with various enzymes and proteins during the peptide synthesis process . The nature of these interactions is primarily chemical, involving the formation and breaking of bonds during the synthesis process .
Cellular Effects
The effects of this compound on cells are primarily observed in the context of peptide synthesis. It influences cell function by contributing to the formation of peptides, which can impact cell signaling pathways, gene expression, and cellular metabolism .
Molecular Mechanism
At the molecular level, this compound exerts its effects through its involvement in peptide synthesis . It can bind to biomolecules, participate in enzyme activation or inhibition, and contribute to changes in gene expression through the peptides it helps synthesize .
Temporal Effects in Laboratory Settings
Over time in laboratory settings, this compound contributes to the synthesis of peptides, which can have long-term effects on cellular function
Metabolic Pathways
This compound is involved in the metabolic pathway of peptide synthesis . It interacts with enzymes involved in this process, and its use can affect metabolic flux and metabolite levels .
Transport and Distribution
In terms of transport and distribution within cells and tissues, this compound is primarily involved in the process of peptide synthesis . It does not appear to interact with transporters or binding proteins, nor does it have known effects on localization or accumulation .
Subcellular Localization
The subcellular localization of this compound is not well-defined as it is a reagent used in peptide synthesis and is not typically found within cells outside of this context .
Vorbereitungsmethoden
Synthetic Routes and Reaction Conditions
The synthesis of Fmoc-Ser(tBu)-OPfp typically involves the following steps:
Protection of Serine: The hydroxyl group of serine is protected using a tert-butyl group to form Fmoc-Ser(tBu)-OH.
Activation of Carboxyl Group: The carboxyl group of Fmoc-Ser(tBu)-OH is activated using pentafluorophenyl trifluoroacetate to form this compound.
The reaction conditions generally involve the use of organic solvents such as dichloromethane and dimethylformamide, with the reactions being carried out at room temperature or slightly elevated temperatures to ensure complete conversion.
Industrial Production Methods
In industrial settings, the production of this compound follows similar synthetic routes but on a larger scale. The process involves the use of automated peptide synthesizers and large-scale reactors to ensure high yield and purity. The use of high-performance liquid chromatography (HPLC) is common for the purification and quality control of the final product.
Analyse Chemischer Reaktionen
Types of Reactions
Fmoc-Ser(tBu)-OPfp undergoes several types of chemical reactions, including:
Substitution Reactions: The pentafluorophenyl ester group is highly reactive and can be substituted by nucleophiles such as amines, leading to the formation of peptide bonds.
Deprotection Reactions: The Fmoc and tBu groups can be removed under specific conditions to expose the serine residue for further reactions.
Common Reagents and Conditions
Substitution Reactions: Common reagents include primary and secondary amines, with reactions typically carried out in organic solvents like
Biologische Aktivität
Fmoc-Ser(tBu)-OPfp, a derivative of serine, is an important compound in the realm of peptide synthesis. It serves as a building block in solid-phase peptide synthesis (SPPS), particularly utilizing the Fmoc (9-fluorenylmethoxycarbonyl) protection strategy. This article explores the biological activity of this compound, including its mechanisms, applications, and relevant research findings.
Chemical Structure and Properties
This compound has the molecular formula C28H24F5NO5 and a molecular weight of approximately 525.49 g/mol. The compound features a fluorenylmethoxycarbonyl group that protects the amino group and a tert-butyl group that protects the hydroxyl side chain of serine. These protective groups are crucial for facilitating controlled reactions during peptide synthesis.
Property | Value |
---|---|
Molecular Formula | C28H24F5NO5 |
Molecular Weight | 525.49 g/mol |
CAS Number | 11180366 |
The mechanism of action for this compound involves the selective protection and deprotection of functional groups during peptide synthesis. The Fmoc group can be removed under mild basic conditions, while the tert-butyl group is cleaved under acidic conditions. This orthogonal protection allows for sequential amino acid addition, making it suitable for synthesizing complex peptides.
Applications in Peptide Synthesis
This compound is widely utilized in various scientific and industrial applications:
- Peptide Synthesis : As a standard serine derivative, it is integral to the synthesis of peptides through SPPS.
- Drug Development : Peptides synthesized using this compound can lead to new therapeutic agents due to the biological relevance of serine in enzyme active sites and signaling pathways.
- Biological Studies : The compound aids in studying protein-protein interactions and enzyme-substrate dynamics.
Self-Assembly Studies
Recent research has shown that Fmoc-Ser(tBu)-OH exhibits self-assembly properties, leading to various morphological structures depending on concentration and temperature conditions. For instance:
- At lower concentrations, flower-like structures form.
- At higher concentrations, these structures transition into elongated rods.
- Heating at elevated temperatures can further alter these morphologies, showcasing potential applications in material science.
Morphological Changes Observed
Concentration Level | Morphological Structure |
---|---|
Low | Flower-like |
High | Long rods |
Heated (70°C) | Transition between rods and flowers |
Case Studies and Research Findings
- Controlled Morphological Changes : A study demonstrated that varying concentrations of Fmoc-Ser(tBu)-OH led to distinct self-assembled structures, indicating its potential use in designing novel materials with specific properties .
- NMR Studies : Nuclear Magnetic Resonance (NMR) spectroscopy confirmed the role of π-π stacking interactions in the self-assembly process, suggesting that these interactions are critical for maintaining structural integrity at different concentrations .
- Biological Activity Implications : Although specific biological activities of this compound have not been extensively documented, its role in synthesizing peptides suggests potential applications in drug development targeting enzyme mechanisms where serine plays a crucial role .
Eigenschaften
IUPAC Name |
(2,3,4,5,6-pentafluorophenyl) (2S)-2-(9H-fluoren-9-ylmethoxycarbonylamino)-3-[(2-methylpropan-2-yl)oxy]propanoate | |
---|---|---|
Source | PubChem | |
URL | https://pubchem.ncbi.nlm.nih.gov | |
Description | Data deposited in or computed by PubChem | |
InChI |
InChI=1S/C28H24F5NO5/c1-28(2,3)38-13-19(26(35)39-25-23(32)21(30)20(29)22(31)24(25)33)34-27(36)37-12-18-16-10-6-4-8-14(16)15-9-5-7-11-17(15)18/h4-11,18-19H,12-13H2,1-3H3,(H,34,36)/t19-/m0/s1 | |
Source | PubChem | |
URL | https://pubchem.ncbi.nlm.nih.gov | |
Description | Data deposited in or computed by PubChem | |
InChI Key |
DOUJYVMLNKRFHE-IBGZPJMESA-N | |
Source | PubChem | |
URL | https://pubchem.ncbi.nlm.nih.gov | |
Description | Data deposited in or computed by PubChem | |
Canonical SMILES |
CC(C)(C)OCC(C(=O)OC1=C(C(=C(C(=C1F)F)F)F)F)NC(=O)OCC2C3=CC=CC=C3C4=CC=CC=C24 | |
Source | PubChem | |
URL | https://pubchem.ncbi.nlm.nih.gov | |
Description | Data deposited in or computed by PubChem | |
Isomeric SMILES |
CC(C)(C)OC[C@@H](C(=O)OC1=C(C(=C(C(=C1F)F)F)F)F)NC(=O)OCC2C3=CC=CC=C3C4=CC=CC=C24 | |
Source | PubChem | |
URL | https://pubchem.ncbi.nlm.nih.gov | |
Description | Data deposited in or computed by PubChem | |
Molecular Formula |
C28H24F5NO5 | |
Source | PubChem | |
URL | https://pubchem.ncbi.nlm.nih.gov | |
Description | Data deposited in or computed by PubChem | |
DSSTOX Substance ID |
DTXSID50457823 | |
Record name | Fmoc-Ser(tBu)-OPfp | |
Source | EPA DSSTox | |
URL | https://comptox.epa.gov/dashboard/DTXSID50457823 | |
Description | DSSTox provides a high quality public chemistry resource for supporting improved predictive toxicology. | |
Molecular Weight |
549.5 g/mol | |
Source | PubChem | |
URL | https://pubchem.ncbi.nlm.nih.gov | |
Description | Data deposited in or computed by PubChem | |
CAS No. |
105751-13-1 | |
Record name | Fmoc-Ser(tBu)-OPfp | |
Source | EPA DSSTox | |
URL | https://comptox.epa.gov/dashboard/DTXSID50457823 | |
Description | DSSTox provides a high quality public chemistry resource for supporting improved predictive toxicology. | |
Retrosynthesis Analysis
AI-Powered Synthesis Planning: Our tool employs the Template_relevance Pistachio, Template_relevance Bkms_metabolic, Template_relevance Pistachio_ringbreaker, Template_relevance Reaxys, Template_relevance Reaxys_biocatalysis model, leveraging a vast database of chemical reactions to predict feasible synthetic routes.
One-Step Synthesis Focus: Specifically designed for one-step synthesis, it provides concise and direct routes for your target compounds, streamlining the synthesis process.
Accurate Predictions: Utilizing the extensive PISTACHIO, BKMS_METABOLIC, PISTACHIO_RINGBREAKER, REAXYS, REAXYS_BIOCATALYSIS database, our tool offers high-accuracy predictions, reflecting the latest in chemical research and data.
Strategy Settings
Precursor scoring | Relevance Heuristic |
---|---|
Min. plausibility | 0.01 |
Model | Template_relevance |
Template Set | Pistachio/Bkms_metabolic/Pistachio_ringbreaker/Reaxys/Reaxys_biocatalysis |
Top-N result to add to graph | 6 |
Feasible Synthetic Routes
Haftungsausschluss und Informationen zu In-Vitro-Forschungsprodukten
Bitte beachten Sie, dass alle Artikel und Produktinformationen, die auf BenchChem präsentiert werden, ausschließlich zu Informationszwecken bestimmt sind. Die auf BenchChem zum Kauf angebotenen Produkte sind speziell für In-vitro-Studien konzipiert, die außerhalb lebender Organismen durchgeführt werden. In-vitro-Studien, abgeleitet von dem lateinischen Begriff "in Glas", beinhalten Experimente, die in kontrollierten Laborumgebungen unter Verwendung von Zellen oder Geweben durchgeführt werden. Es ist wichtig zu beachten, dass diese Produkte nicht als Arzneimittel oder Medikamente eingestuft sind und keine Zulassung der FDA für die Vorbeugung, Behandlung oder Heilung von medizinischen Zuständen, Beschwerden oder Krankheiten erhalten haben. Wir müssen betonen, dass jede Form der körperlichen Einführung dieser Produkte in Menschen oder Tiere gesetzlich strikt untersagt ist. Es ist unerlässlich, sich an diese Richtlinien zu halten, um die Einhaltung rechtlicher und ethischer Standards in Forschung und Experiment zu gewährleisten.