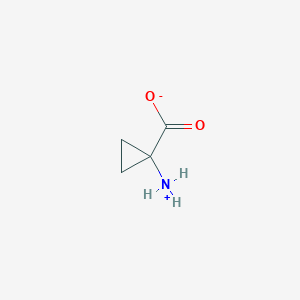
1-Aminocyclopropancarbonsäure
Übersicht
Beschreibung
1-Aminocyclopropan-1-carbonsäure ist eine disubstituierte cyclische Alpha-Aminosäure, bei der ein Cyclopropanring mit dem Alpha-Kohlenstoffatom der Aminosäure verschmolzen ist. Sie ist ein weißer Feststoff und kommt natürlicherweise in verschiedenen biologischen Systemen vor .
Wissenschaftliche Forschungsanwendungen
1-Aminocyclopropan-1-carbonsäure hat zahlreiche Anwendungen in der wissenschaftlichen Forschung:
Chemie: Sie wird als Zwischenprodukt bei der Synthese verschiedener organischer Verbindungen verwendet.
Industrie: Sie wird bei der Herstellung bestimmter Pharmazeutika und Agrochemikalien verwendet.
5. Wirkmechanismus
1-Aminocyclopropan-1-carbonsäure übt seine Wirkungen durch die Modulation der Aktivität von NMDA-Rezeptoren aus. Sie wirkt als partieller Agonist an der Glycin-Bindungsstelle an NMDA-Rezeptoren in Gegenwart niedriger Glutamatspiegel und als kompetitiver Antagonist an der Glutamat-Bindungsstelle mit hohen Glutamatspiegeln. Diese Doppelfunktion trägt zum Schutz vor Neuronenzellabsterben und zur Abschwächung der exzessiven NMDA-Rezeptor-Signalgebung bei, die zu Neurotoxizität führen kann .
Wirkmechanismus
Target of Action
1-Aminocyclopropanecarboxylic Acid (ACC) is the direct precursor of the plant hormone ethylene . It is synthesized from S-adenosyl-L-methionine (SAM) by ACC synthases (ACSs) and subsequently oxidized to ethylene by ACC oxidases (ACOs) . ACC also acts as an agonist of the N-methyl-D-aspartate (NMDA) receptor .
Mode of Action
ACC’s interaction with its targets results in a wide range of effects. As a precursor to ethylene, ACC plays a crucial role in the biosynthesis of this plant hormone . Additionally, ACC signaling promotes secretion of the pollen tube chemoattractant LURE1.2 in ovular sporophytic tissue, enhancing pollen tube attraction . It also activates Ca 2+ -containing ion currents via glutamate receptor-like (GLR) channels in root protoplasts .
Biochemical Pathways
ACC is involved in the ethylene biosynthesis pathway. Its biosynthesis starts with the conversion of the amino acid methionine to S-adenosyl L-methionine (SAM) by SAM synthetase. SAM is then converted to ACC by ACC synthase (ACS). The by-product of this reaction, 5’-methylthioadenosine (MTA), is recycled back into the Yang cycle while ACC is oxidized to ethylene by ACC oxidase (ACO) .
Pharmacokinetics
It is known that acc can be used by soil microorganisms (both bacteria and fungi) as a source of nitrogen and carbon .
Result of Action
The action of ACC results in a variety of molecular and cellular effects. As a precursor to ethylene, ACC influences a wide range of developmental processes and responses to biotic and abiotic stresses . As an agonist of the NMDA receptor, ACC elicits protection against glutamate-induced neurotoxicity in neurons .
Action Environment
Environmental factors can influence the action, efficacy, and stability of ACC. For example, using ACC to incubate soils has been proven to induce the gene abundance encoding ACC-deaminases, which may have positive consequences on plant growth and stress tolerance .
Biochemische Analyse
Biochemical Properties
1-Aminocyclopropanecarboxylic acid plays a significant role in biochemical reactions. It is the precursor to the plant hormone ethylene . It is synthesized by the enzyme ACC synthase (EC 4.4.1.14) from methionine and converted to ethylene by ACC oxidase (EC 1.14.17.4) .
Cellular Effects
1-Aminocyclopropanecarboxylic acid influences cell function by promoting secretion of the pollen tube chemoattractant LURE1.2 in ovular sporophytic tissue, enhancing pollen tube attraction . It also activates Ca 2+ -containing ion currents via glutamate receptor-like (GLR) channels in root protoplasts .
Molecular Mechanism
The molecular mechanism of 1-Aminocyclopropanecarboxylic acid involves its role as a partial agonist acting at the N-methyl-D-aspartate (NMDA) receptor glycine modulatory site . It also plays a vital role in plant development .
Metabolic Pathways
1-Aminocyclopropanecarboxylic acid is involved in the metabolic pathway of the plant hormone ethylene . It interacts with enzymes such as ACC synthase and ACC oxidase .
Vorbereitungsmethoden
1-Aminocyclopropan-1-carbonsäure kann durch verschiedene Verfahren synthetisiert werden. Ein übliches Verfahren beinhaltet die Reaktion von Cyclopropan mit Ammoniak und Kohlendioxid unter Hochdruck- und Hochtemperaturbedingungen. Eine andere Methode beinhaltet die Verwendung von Cyclopropancarbonsäure als Vorläufer, die dann zu 1-Aminocyclopropan-1-carbonsäure aminiert wird .
Analyse Chemischer Reaktionen
1-Aminocyclopropan-1-carbonsäure unterliegt verschiedenen chemischen Reaktionen, darunter:
Oxidation: Sie kann zu entsprechenden Oxoverbindungen oxidiert werden.
Reduktion: Reduktionsreaktionen können sie in verschiedene Aminderivate umwandeln.
Vergleich Mit ähnlichen Verbindungen
1-Aminocyclopropan-1-carbonsäure ist aufgrund ihrer cyclischen Struktur und ihrer Doppelfunktion an NMDA-Rezeptoren einzigartig. Ähnliche Verbindungen umfassen:
Cyclopropancarbonsäure: Wird als Vorläufer bei der Synthese von 1-Aminocyclopropan-1-carbonsäure verwendet.
Ethylen: Ein Pflanzenhormon, das aus 1-Aminocyclopropan-1-carbonsäure synthetisiert wird.
Andere cyclopropan-substituierte Aminosäuren: Diese Verbindungen weisen strukturelle Ähnlichkeiten auf, unterscheiden sich jedoch in ihren biologischen Aktivitäten und Anwendungen.
Biologische Aktivität
1-Aminocyclopropanecarboxylic acid (ACPC) is a compound that has garnered attention for its diverse biological activities, particularly in the fields of plant biology and neuroprotection. This article explores its biological activity, mechanisms of action, and potential applications based on recent research findings.
Overview of 1-Aminocyclopropanecarboxylic Acid
1-Aminocyclopropanecarboxylic acid is structurally related to 1-aminocyclopropane-1-carboxylic acid (ACC), a well-known precursor to ethylene in plants. While ACC is primarily recognized for its role in plant growth and development, ACPC has been identified as having significant neuroprotective properties and potential therapeutic applications in cardiovascular health.
Ethylene Production in Plants
ACPC has been used as a proxy for ethylene in various studies. It is readily converted to ethylene by plant tissues, influencing numerous physiological processes such as fruit ripening, leaf senescence, and stress responses. The role of ACC in these processes has been extensively documented:
- Ethylene Synthesis : ACC serves as a key precursor in ethylene biosynthesis, which is crucial for plant growth regulation and response to environmental stimuli .
- Transport Mechanisms : In Arabidopsis thaliana, ACC is transported by specific amino acid transporters, facilitating its uptake and conversion to ethylene .
Neuroprotective Effects
ACPC exhibits neuroprotective properties by acting as an antagonist to N-methyl-D-aspartate (NMDA) receptors, which are implicated in excitotoxicity associated with various neurological disorders:
- Reduction of Neurotoxicity : Studies have shown that ACPC can protect neurons from glutamate-induced neurotoxicity by reducing NMDA receptor activation. This effect is particularly relevant in models of stroke-prone spontaneously hypertensive rats, where ACPC administration resulted in lower blood pressure and reduced mortality rates from stroke .
- Antioxidant Activity : ACPC has been linked to the induction of heme oxygenase-1 (HO-1), an enzyme with antioxidant properties that contributes to vascular relaxation and protection against oxidative stress .
Plant Studies
A study demonstrated that beneficial rhizobacteria producing ACC deaminase could utilize ACC as a nitrogen source, thereby promoting plant growth under stress conditions. The study quantified the degradation of ACC by various bacterial isolates, highlighting their potential role in enhancing plant resilience .
Bacterial Isolate | ACC Degradation (%) | IAA Production (µg/ml) | Biofilm Formation |
---|---|---|---|
4F1 | 45.36 | 56.69 | High |
11G | 45.03 | 50.12 | Moderate |
20B | 36.72 | 45.00 | Moderate |
Neuroprotective Studies
In a controlled study involving stroke-prone rats, ACPC was administered at a dose of 50 mg/kg per day for four weeks. The results indicated significant improvements in cardiovascular parameters and reductions in markers of oxidative stress:
- Systolic Blood Pressure : Significantly lower in the ACPC group compared to controls.
- Oxidative Stress Markers : Decreased expression of nitrotyrosine and reduced levels of oxidative damage products such as 8-OHdG were observed .
Case Studies
- Plant Stress Response : In Arabidopsis, mutants deficient in ACC uptake showed severe growth defects when exposed to nitrogen-limited conditions, underscoring the importance of ACC in nutrient uptake and stress response mechanisms .
- Cardiovascular Protection : A clinical study indicated that ACPC's modulation of NMDA receptor activity could have implications for treating conditions like hypertension and stroke, suggesting its potential as a therapeutic agent .
Eigenschaften
IUPAC Name |
1-aminocyclopropane-1-carboxylic acid | |
---|---|---|
Source | PubChem | |
URL | https://pubchem.ncbi.nlm.nih.gov | |
Description | Data deposited in or computed by PubChem | |
InChI |
InChI=1S/C4H7NO2/c5-4(1-2-4)3(6)7/h1-2,5H2,(H,6,7) | |
Source | PubChem | |
URL | https://pubchem.ncbi.nlm.nih.gov | |
Description | Data deposited in or computed by PubChem | |
InChI Key |
PAJPWUMXBYXFCZ-UHFFFAOYSA-N | |
Source | PubChem | |
URL | https://pubchem.ncbi.nlm.nih.gov | |
Description | Data deposited in or computed by PubChem | |
Canonical SMILES |
C1CC1(C(=O)O)N | |
Source | PubChem | |
URL | https://pubchem.ncbi.nlm.nih.gov | |
Description | Data deposited in or computed by PubChem | |
Molecular Formula |
C4H7NO2 | |
Record name | 1-Aminocyclopropanecarboxylic acid | |
Source | Wikipedia | |
URL | https://en.wikipedia.org/wiki/1-Aminocyclopropanecarboxylic_acid | |
Description | Chemical information link to Wikipedia. | |
Source | PubChem | |
URL | https://pubchem.ncbi.nlm.nih.gov | |
Description | Data deposited in or computed by PubChem | |
DSSTOX Substance ID |
DTXSID9039577 | |
Record name | 1-Aminocyclopropane-1-carboxylic acid | |
Source | EPA DSSTox | |
URL | https://comptox.epa.gov/dashboard/DTXSID9039577 | |
Description | DSSTox provides a high quality public chemistry resource for supporting improved predictive toxicology. | |
Molecular Weight |
101.10 g/mol | |
Source | PubChem | |
URL | https://pubchem.ncbi.nlm.nih.gov | |
Description | Data deposited in or computed by PubChem | |
Physical Description |
Solid | |
Record name | 1-Aminocyclopropanecarboxylic acid | |
Source | Human Metabolome Database (HMDB) | |
URL | http://www.hmdb.ca/metabolites/HMDB0036458 | |
Description | The Human Metabolome Database (HMDB) is a freely available electronic database containing detailed information about small molecule metabolites found in the human body. | |
Explanation | HMDB is offered to the public as a freely available resource. Use and re-distribution of the data, in whole or in part, for commercial purposes requires explicit permission of the authors and explicit acknowledgment of the source material (HMDB) and the original publication (see the HMDB citing page). We ask that users who download significant portions of the database cite the HMDB paper in any resulting publications. | |
CAS No. |
22059-21-8 | |
Record name | 1-Aminocyclopropane-1-carboxylic acid | |
Source | CAS Common Chemistry | |
URL | https://commonchemistry.cas.org/detail?cas_rn=22059-21-8 | |
Description | CAS Common Chemistry is an open community resource for accessing chemical information. Nearly 500,000 chemical substances from CAS REGISTRY cover areas of community interest, including common and frequently regulated chemicals, and those relevant to high school and undergraduate chemistry classes. This chemical information, curated by our expert scientists, is provided in alignment with our mission as a division of the American Chemical Society. | |
Explanation | The data from CAS Common Chemistry is provided under a CC-BY-NC 4.0 license, unless otherwise stated. | |
Record name | 1-Aminocyclopropane-1-carboxylic acid | |
Source | ChemIDplus | |
URL | https://pubchem.ncbi.nlm.nih.gov/substance/?source=chemidplus&sourceid=0022059218 | |
Description | ChemIDplus is a free, web search system that provides access to the structure and nomenclature authority files used for the identification of chemical substances cited in National Library of Medicine (NLM) databases, including the TOXNET system. | |
Record name | 1-Aminocyclopropanecarboxylic Acid | |
Source | DrugBank | |
URL | https://www.drugbank.ca/drugs/DB02085 | |
Description | The DrugBank database is a unique bioinformatics and cheminformatics resource that combines detailed drug (i.e. chemical, pharmacological and pharmaceutical) data with comprehensive drug target (i.e. sequence, structure, and pathway) information. | |
Explanation | Creative Common's Attribution-NonCommercial 4.0 International License (http://creativecommons.org/licenses/by-nc/4.0/legalcode) | |
Record name | 22059-21-8 | |
Source | DTP/NCI | |
URL | https://dtp.cancer.gov/dtpstandard/servlet/dwindex?searchtype=NSC&outputformat=html&searchlist=98430 | |
Description | The NCI Development Therapeutics Program (DTP) provides services and resources to the academic and private-sector research communities worldwide to facilitate the discovery and development of new cancer therapeutic agents. | |
Explanation | Unless otherwise indicated, all text within NCI products is free of copyright and may be reused without our permission. Credit the National Cancer Institute as the source. | |
Record name | 1-Aminocyclopropane-1-carboxylic acid | |
Source | EPA DSSTox | |
URL | https://comptox.epa.gov/dashboard/DTXSID9039577 | |
Description | DSSTox provides a high quality public chemistry resource for supporting improved predictive toxicology. | |
Record name | 1-AMINOCYCLOPROPANECARBOXYLIC ACID | |
Source | FDA Global Substance Registration System (GSRS) | |
URL | https://gsrs.ncats.nih.gov/ginas/app/beta/substances/3K9EJ633GL | |
Description | The FDA Global Substance Registration System (GSRS) enables the efficient and accurate exchange of information on what substances are in regulated products. Instead of relying on names, which vary across regulatory domains, countries, and regions, the GSRS knowledge base makes it possible for substances to be defined by standardized, scientific descriptions. | |
Explanation | Unless otherwise noted, the contents of the FDA website (www.fda.gov), both text and graphics, are not copyrighted. They are in the public domain and may be republished, reprinted and otherwise used freely by anyone without the need to obtain permission from FDA. Credit to the U.S. Food and Drug Administration as the source is appreciated but not required. | |
Record name | 1-Aminocyclopropanecarboxylic acid | |
Source | Human Metabolome Database (HMDB) | |
URL | http://www.hmdb.ca/metabolites/HMDB0036458 | |
Description | The Human Metabolome Database (HMDB) is a freely available electronic database containing detailed information about small molecule metabolites found in the human body. | |
Explanation | HMDB is offered to the public as a freely available resource. Use and re-distribution of the data, in whole or in part, for commercial purposes requires explicit permission of the authors and explicit acknowledgment of the source material (HMDB) and the original publication (see the HMDB citing page). We ask that users who download significant portions of the database cite the HMDB paper in any resulting publications. | |
Melting Point |
229 - 231 °C | |
Record name | 1-Aminocyclopropanecarboxylic acid | |
Source | Human Metabolome Database (HMDB) | |
URL | http://www.hmdb.ca/metabolites/HMDB0036458 | |
Description | The Human Metabolome Database (HMDB) is a freely available electronic database containing detailed information about small molecule metabolites found in the human body. | |
Explanation | HMDB is offered to the public as a freely available resource. Use and re-distribution of the data, in whole or in part, for commercial purposes requires explicit permission of the authors and explicit acknowledgment of the source material (HMDB) and the original publication (see the HMDB citing page). We ask that users who download significant portions of the database cite the HMDB paper in any resulting publications. | |
Retrosynthesis Analysis
AI-Powered Synthesis Planning: Our tool employs the Template_relevance Pistachio, Template_relevance Bkms_metabolic, Template_relevance Pistachio_ringbreaker, Template_relevance Reaxys, Template_relevance Reaxys_biocatalysis model, leveraging a vast database of chemical reactions to predict feasible synthetic routes.
One-Step Synthesis Focus: Specifically designed for one-step synthesis, it provides concise and direct routes for your target compounds, streamlining the synthesis process.
Accurate Predictions: Utilizing the extensive PISTACHIO, BKMS_METABOLIC, PISTACHIO_RINGBREAKER, REAXYS, REAXYS_BIOCATALYSIS database, our tool offers high-accuracy predictions, reflecting the latest in chemical research and data.
Strategy Settings
Precursor scoring | Relevance Heuristic |
---|---|
Min. plausibility | 0.01 |
Model | Template_relevance |
Template Set | Pistachio/Bkms_metabolic/Pistachio_ringbreaker/Reaxys/Reaxys_biocatalysis |
Top-N result to add to graph | 6 |
Feasible Synthetic Routes
A: 1-Aminocyclopropanecarboxylic acid (ACPC) acts as a high-affinity partial agonist at the strychnine-insensitive glycine recognition site associated with the N-methyl-D-aspartate (NMDA) subtype of glutamate receptors. [] This interaction is crucial because the occupation of these glycine sites is required for the proper functioning of NMDA receptor-coupled cation channels. [] As a partial agonist, ACPC can both stimulate and inhibit NMDA receptor activity depending on its concentration and the presence of other ligands like glutamate. [, ] This dual action contributes to its neuroprotective effects by reducing excessive NMDA receptor activation and excitotoxicity. [, , ]
ANone: While the provided research articles don't explicitly detail all spectroscopic data, we can confirm:
- Spectroscopic Data: For specific spectroscopic data (NMR, IR, etc.), refer to individual publications focused on ACPC synthesis or characterization. [, ]
ANone: The provided research focuses primarily on ACPC's biological activity. Information regarding its material compatibility and stability under various conditions (temperature, pH, solvents) is limited within these articles.
A: The provided research primarily focuses on ACPC's role as a ligand for the NMDA receptor. While ACPC is derived from 1-aminocyclopropanecarboxylic acid, a precursor in ethylene biosynthesis in plants, [, , ] the provided articles do not elaborate on its direct catalytic properties or applications in chemical reactions.
ANone: While the research highlights ACPC's potential therapeutic benefits, the articles provided do not delve into detailed computational chemistry studies, simulations, QSAR models, or structure-activity relationship analyses.
A: While not extensively explored in these articles, modifications to the ACPC structure, particularly substitutions on the cyclopropane ring, can influence its conformational properties and potentially impact its interaction with the NMDA receptor. [, , , ] Further research is needed to fully elucidate the SAR of ACPC and design analogs with improved potency and selectivity.
ANone: The provided research focuses on the scientific and pharmacological aspects of ACPC. It does not discuss SHE (Safety, Health, and Environment) regulations or compliance information.
A: Research indicates that ACPC exhibits favorable pharmacokinetic properties across multiple species, including mice, rats, monkeys, dogs, and humans. [] Its volume of distribution remains consistent across these species, and its clearance aligns with glomerular filtration rates, except in dogs where tubular reabsorption plays a role. [] Notably, allometric relationships for ACPC clearance and half-life show predictability across these species. []
ANone: ACPC has demonstrated neuroprotective effects both in vitro and in vivo:
- In vitro: ACPC effectively protects cerebellar granule cells from glutamate-induced neurotoxicity, a key mechanism implicated in neurodegenerative diseases. [, , ] It achieves this by reducing NMDA-stimulated increases in cGMP levels and intracellular calcium concentrations ([Ca+2]i), thus mitigating excitotoxic damage. [, ]
- In vivo: In gerbil models of forebrain ischemia, ACPC administration significantly improved survival rates and protected hippocampal CA1 neurons from ischemic damage. [] Moreover, in stroke-prone spontaneously hypertensive rats (SHRSP), chronic ACPC treatment normalized blood pressure, reduced stroke mortality, and increased plasma superoxide dismutase (SOD) activity, suggesting antioxidant and vascular protective effects. [] These findings were corroborated by decreased levels of oxidative stress markers like nitrotyrosine and 8-hydroxy-2′-deoxyguanosine (8-OHdG) in the brain. []
Haftungsausschluss und Informationen zu In-Vitro-Forschungsprodukten
Bitte beachten Sie, dass alle Artikel und Produktinformationen, die auf BenchChem präsentiert werden, ausschließlich zu Informationszwecken bestimmt sind. Die auf BenchChem zum Kauf angebotenen Produkte sind speziell für In-vitro-Studien konzipiert, die außerhalb lebender Organismen durchgeführt werden. In-vitro-Studien, abgeleitet von dem lateinischen Begriff "in Glas", beinhalten Experimente, die in kontrollierten Laborumgebungen unter Verwendung von Zellen oder Geweben durchgeführt werden. Es ist wichtig zu beachten, dass diese Produkte nicht als Arzneimittel oder Medikamente eingestuft sind und keine Zulassung der FDA für die Vorbeugung, Behandlung oder Heilung von medizinischen Zuständen, Beschwerden oder Krankheiten erhalten haben. Wir müssen betonen, dass jede Form der körperlichen Einführung dieser Produkte in Menschen oder Tiere gesetzlich strikt untersagt ist. Es ist unerlässlich, sich an diese Richtlinien zu halten, um die Einhaltung rechtlicher und ethischer Standards in Forschung und Experiment zu gewährleisten.