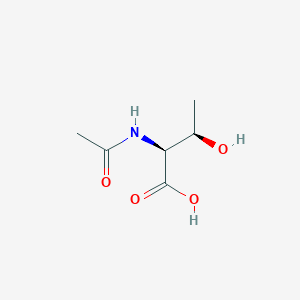
N-Acetylthreonin
Übersicht
Beschreibung
N-Acetylthreonine belongs to the class of organic compounds known as n-acyl-l-alpha-amino acids. These are n-acylated alpha amino acids which have the L-configuration of the alpha-carbon atom. N-Acetylthreonine has been primarily detected in feces.
N-acetyl-L-threonine is a N-acetyl-L-amino acid that is the N-acetyl derivative of L-threonine. It is a L-threonine derivative and a N-acetyl-L-amino acid. It is a conjugate acid of a N-acetyl-L-threoninate.
Wissenschaftliche Forschungsanwendungen
Sequenzierung und Entblockierung von Proteinen
N-Acetylthreonin ist an der Entblockierung von N-terminal modifizierten Proteinen beteiligt, einem entscheidenden Schritt bei der Proteinsequenzierung. Dieser Prozess ist für Proteine essentiell, die mit Acyl- und Alkylgruppen blockiert sind, was eine direkte Edman-Degradation verhindert .
Proteolytischer Abbau
Es kann durch eine N-Acylpeptidhydrolase aus Peptiden freigesetzt werden, die während des proteolytischen Abbaus gebildet werden, der Teil des Proteinstoffwechsels ist .
Metabolom-Profiling
This compound erscheint in metabolomischen Profilstudien, die niedermolekulare zirkulierende Metaboliten in verschiedenen biologischen Proben analysieren .
Biochemische Analyse
Diese Verbindung wird auch in der globalen biochemischen Analyse von Plasma, Serum und Vollblut verwendet, um verschiedene Metaboliten und ihre Auswirkungen auf die menschliche Gesundheit zu untersuchen .
Wirkmechanismus
Target of Action
N-Acetylthreonine, also known as Ac-thr-oh, is thought to exert its mechanism of action through the enzyme threonine acetyltransferase . This enzyme is responsible for catalyzing threonine’s acetylation process . It is also associated with serum- and glucocorticoid-inducible kinase 1 , a serine/threonine protein kinase that modulates angiotensin II and sodium reabsorption in tubular cells .
Mode of Action
The compound interacts with its targets, leading to the acetylation of threonine, a process catalyzed by threonine acetyltransferase . This interaction results in the modulation of angiotensin II and sodium reabsorption in tubular cells, contributing to fluid retention and hypertension, enhanced coagulation, and increased deposition of matrix proteins .
Biochemical Pathways
N-Acetylthreonine is a product of threonine metabolism . The acetylation of threonine can occur through the action of N-acylpeptide hydrolase from peptides generated by proteolytic degradation . This process plays a role in regulating glucose and lipid metabolism, as well as influencing the immune system’s modulation .
Pharmacokinetics
It is known to be soluble in water , which may influence its absorption, distribution, metabolism, and excretion (ADME) properties
Result of Action
The action of N-Acetylthreonine leads to the modulation of angiotensin II and sodium reabsorption in tubular cells . This can contribute to fluid retention and hypertension, enhanced coagulation, and increased deposition of matrix proteins . It also influences the immune system’s modulation .
Action Environment
The action, efficacy, and stability of N-Acetylthreonine can be influenced by various environmental factors. For instance, its solubility in water may affect its absorption and distribution in the body.
Biochemische Analyse
Biochemical Properties
These compounds are N-acylated alpha amino acids which have the L-configuration at the alpha-carbon atom . N-Acetylthreonine is considered to be soluble (in water) and acidic .
Molecular Mechanism
N-Acetylthreonine is thought to exert its mechanism of action through the enzyme threonine acetyltransferase, responsible for catalyzing threonine’s acetylation process . This enzyme plays a role in regulating glucose and lipid metabolism, as well as influencing the immune system’s modulation .
Metabolic Pathways
N-Acetylthreonine is involved in various metabolic pathways. It’s associated with circulating metabolites in various metabolic pathways, particularly lipids, amino acids, and cofactors/vitamins .
Eigenschaften
IUPAC Name |
(2S,3R)-2-acetamido-3-hydroxybutanoic acid | |
---|---|---|
Source | PubChem | |
URL | https://pubchem.ncbi.nlm.nih.gov | |
Description | Data deposited in or computed by PubChem | |
InChI |
InChI=1S/C6H11NO4/c1-3(8)5(6(10)11)7-4(2)9/h3,5,8H,1-2H3,(H,7,9)(H,10,11)/t3-,5+/m1/s1 | |
Source | PubChem | |
URL | https://pubchem.ncbi.nlm.nih.gov | |
Description | Data deposited in or computed by PubChem | |
InChI Key |
PEDXUVCGOLSNLQ-WUJLRWPWSA-N | |
Source | PubChem | |
URL | https://pubchem.ncbi.nlm.nih.gov | |
Description | Data deposited in or computed by PubChem | |
Canonical SMILES |
CC(C(C(=O)O)NC(=O)C)O | |
Source | PubChem | |
URL | https://pubchem.ncbi.nlm.nih.gov | |
Description | Data deposited in or computed by PubChem | |
Isomeric SMILES |
C[C@H]([C@@H](C(=O)O)NC(=O)C)O | |
Source | PubChem | |
URL | https://pubchem.ncbi.nlm.nih.gov | |
Description | Data deposited in or computed by PubChem | |
Molecular Formula |
C6H11NO4 | |
Source | PubChem | |
URL | https://pubchem.ncbi.nlm.nih.gov | |
Description | Data deposited in or computed by PubChem | |
DSSTOX Substance ID |
DTXSID80168975 | |
Record name | N-Acetylthreonine | |
Source | EPA DSSTox | |
URL | https://comptox.epa.gov/dashboard/DTXSID80168975 | |
Description | DSSTox provides a high quality public chemistry resource for supporting improved predictive toxicology. | |
Molecular Weight |
161.16 g/mol | |
Source | PubChem | |
URL | https://pubchem.ncbi.nlm.nih.gov | |
Description | Data deposited in or computed by PubChem | |
CAS No. |
17093-74-2 | |
Record name | N-Acetyl-L-threonine | |
Source | CAS Common Chemistry | |
URL | https://commonchemistry.cas.org/detail?cas_rn=17093-74-2 | |
Description | CAS Common Chemistry is an open community resource for accessing chemical information. Nearly 500,000 chemical substances from CAS REGISTRY cover areas of community interest, including common and frequently regulated chemicals, and those relevant to high school and undergraduate chemistry classes. This chemical information, curated by our expert scientists, is provided in alignment with our mission as a division of the American Chemical Society. | |
Explanation | The data from CAS Common Chemistry is provided under a CC-BY-NC 4.0 license, unless otherwise stated. | |
Record name | N-Acetylthreonine | |
Source | ChemIDplus | |
URL | https://pubchem.ncbi.nlm.nih.gov/substance/?source=chemidplus&sourceid=0017093742 | |
Description | ChemIDplus is a free, web search system that provides access to the structure and nomenclature authority files used for the identification of chemical substances cited in National Library of Medicine (NLM) databases, including the TOXNET system. | |
Record name | N-Acetylthreonine | |
Source | EPA DSSTox | |
URL | https://comptox.epa.gov/dashboard/DTXSID80168975 | |
Description | DSSTox provides a high quality public chemistry resource for supporting improved predictive toxicology. | |
Record name | N-ACETYLTHREONINE | |
Source | FDA Global Substance Registration System (GSRS) | |
URL | https://gsrs.ncats.nih.gov/ginas/app/beta/substances/V0E98V3R9O | |
Description | The FDA Global Substance Registration System (GSRS) enables the efficient and accurate exchange of information on what substances are in regulated products. Instead of relying on names, which vary across regulatory domains, countries, and regions, the GSRS knowledge base makes it possible for substances to be defined by standardized, scientific descriptions. | |
Explanation | Unless otherwise noted, the contents of the FDA website (www.fda.gov), both text and graphics, are not copyrighted. They are in the public domain and may be republished, reprinted and otherwise used freely by anyone without the need to obtain permission from FDA. Credit to the U.S. Food and Drug Administration as the source is appreciated but not required. | |
Record name | N-Acetylthreonine | |
Source | Human Metabolome Database (HMDB) | |
URL | http://www.hmdb.ca/metabolites/HMDB0062557 | |
Description | The Human Metabolome Database (HMDB) is a freely available electronic database containing detailed information about small molecule metabolites found in the human body. | |
Explanation | HMDB is offered to the public as a freely available resource. Use and re-distribution of the data, in whole or in part, for commercial purposes requires explicit permission of the authors and explicit acknowledgment of the source material (HMDB) and the original publication (see the HMDB citing page). We ask that users who download significant portions of the database cite the HMDB paper in any resulting publications. | |
Retrosynthesis Analysis
AI-Powered Synthesis Planning: Our tool employs the Template_relevance Pistachio, Template_relevance Bkms_metabolic, Template_relevance Pistachio_ringbreaker, Template_relevance Reaxys, Template_relevance Reaxys_biocatalysis model, leveraging a vast database of chemical reactions to predict feasible synthetic routes.
One-Step Synthesis Focus: Specifically designed for one-step synthesis, it provides concise and direct routes for your target compounds, streamlining the synthesis process.
Accurate Predictions: Utilizing the extensive PISTACHIO, BKMS_METABOLIC, PISTACHIO_RINGBREAKER, REAXYS, REAXYS_BIOCATALYSIS database, our tool offers high-accuracy predictions, reflecting the latest in chemical research and data.
Strategy Settings
Precursor scoring | Relevance Heuristic |
---|---|
Min. plausibility | 0.01 |
Model | Template_relevance |
Template Set | Pistachio/Bkms_metabolic/Pistachio_ringbreaker/Reaxys/Reaxys_biocatalysis |
Top-N result to add to graph | 6 |
Feasible Synthetic Routes
Q1: What is the biological significance of N-acetylthreonine and how does its presence in circulation relate to renal function?
A1: N-acetylthreonine is a modified amino acid that has emerged as a potential biomarker for declining renal function, especially in patients with diabetes. While its exact biological role remains unclear, studies suggest it may reflect altered amino acid metabolism and tubular injury. For instance, in a prospective study involving patients with type 1 diabetes and chronic kidney disease, elevated serum levels of N-acetylthreonine were linked to a faster decline in renal function and an increased risk of end-stage renal disease [, ]. These associations were independent of traditional risk factors, highlighting its potential as a prognostic indicator.
Q2: Can metabolomics be used to predict the progression of renal dysfunction in patients with type 2 diabetes?
A2: Yes, metabolomics shows promise in predicting renal decline in type 2 diabetes. A study using gas chromatography/mass spectroscopy and ultra-high performance liquid chromatography/mass spectrometry identified a panel of serum metabolites, including N-acetylthreonine, that could predict both declining estimated glomerular filtration rate (eGFR) and increased albuminuria in these patients []. This suggests that metabolic profiling could enhance risk stratification and early intervention strategies for diabetic kidney disease.
Q3: How does N-acetylthreonine relate to muscle metabolism and the impact of interventions like high-protein diets and neuromuscular electrical stimulation?
A3: Research indicates a potential link between N-acetylthreonine, muscle metabolism, and interventions like high-protein diets combined with neuromuscular electrical stimulation (HPRO+NMES). In a study examining the effects of HPRO+NMES on patients recovering from aneurysmal subarachnoid hemorrhage, N-acetylthreonine emerged as one of the key metabolites associated with protein intake and muscle volume preservation []. This suggests that N-acetylthreonine may be involved in the metabolic pathways influenced by HPRO+NMES, potentially contributing to its beneficial effects on muscle health.
Q4: Does physical activity influence the levels of N-acetylthreonine in the body?
A4: Yes, research indicates a strong association between physical activity and N-acetylthreonine levels. A study utilizing doubly labeled water, accelerometers, and dietary recalls to assess physical activity levels found that higher physical activity was associated with altered levels of 164 metabolites, including N-acetylthreonine []. This underscores the broad influence of physical activity on metabolic pathways and suggests a potential role for N-acetylthreonine in mediating some of these effects.
Q5: Can N-acetylthreonine levels be used to assess disease severity and clinical behavior in patients with pulmonary sarcoidosis?
A5: Preliminary research suggests a potential link between N-acetylthreonine levels and pulmonary sarcoidosis. A metabolomics study on sarcoidosis patients identified N-acetylthreonine as one of the amino acids differentially expressed in the plasma of these individuals compared to controls []. Furthermore, N-acetylthreonine levels showed a correlation with forced vital capacity, a measure of lung function, in these patients. While further research is needed to validate these findings, this suggests a potential role for N-acetylthreonine as a biomarker in understanding and managing sarcoidosis.
Q6: Is there a connection between N-acetylthreonine and breast cancer risk?
A6: Emerging evidence suggests a potential link between N-acetylthreonine and breast cancer risk, possibly mediated by physical activity. A study examining the association between physical activity-associated metabolites and breast cancer risk found that N-acetylthreonine, along with other metabolites, was associated with a reduced risk of postmenopausal breast cancer []. This suggests that N-acetylthreonine, potentially influenced by physical activity, could play a role in the metabolic pathways involved in breast cancer development.
Haftungsausschluss und Informationen zu In-Vitro-Forschungsprodukten
Bitte beachten Sie, dass alle Artikel und Produktinformationen, die auf BenchChem präsentiert werden, ausschließlich zu Informationszwecken bestimmt sind. Die auf BenchChem zum Kauf angebotenen Produkte sind speziell für In-vitro-Studien konzipiert, die außerhalb lebender Organismen durchgeführt werden. In-vitro-Studien, abgeleitet von dem lateinischen Begriff "in Glas", beinhalten Experimente, die in kontrollierten Laborumgebungen unter Verwendung von Zellen oder Geweben durchgeführt werden. Es ist wichtig zu beachten, dass diese Produkte nicht als Arzneimittel oder Medikamente eingestuft sind und keine Zulassung der FDA für die Vorbeugung, Behandlung oder Heilung von medizinischen Zuständen, Beschwerden oder Krankheiten erhalten haben. Wir müssen betonen, dass jede Form der körperlichen Einführung dieser Produkte in Menschen oder Tiere gesetzlich strikt untersagt ist. Es ist unerlässlich, sich an diese Richtlinien zu halten, um die Einhaltung rechtlicher und ethischer Standards in Forschung und Experiment zu gewährleisten.