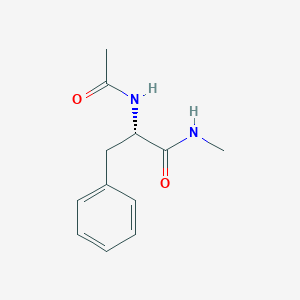
Ac-Phe-NHMe
Übersicht
Beschreibung
Ac-Phe-NHMe is a chiral compound with a specific three-dimensional arrangement of atoms
Wirkmechanismus
Target of Action
Ac-Phe-NHMe, also known as Acetyl-L-phenylalanine methyl amide or (S)-2-Acetamido-N-methyl-3-phenylpropanamide, is a dipeptide model that has been studied for its structural properties It’s known to form structures such as β-sheet related configurations or hydrogen-bonded structures .
Mode of Action
The interaction of this compound with its targets involves the formation of specific structural arrangements. Three isomers of the this compound monomer have been identified, which contain either a β-sheet related configuration or hydrogen-bonded structures . The most prominent species has a β-sheet related conformation . These interactions result in changes in the structural arrangements of the compound.
Biochemical Pathways
The compound is known to form β-sheet model systems in the gas phase . This suggests that it may influence protein folding and other processes involving β-sheet structures.
Result of Action
The molecular and cellular effects of this compound’s action primarily involve changes in structural arrangements. The compound forms β-sheet related configurations or hydrogen-bonded structures . These structures could potentially influence protein folding and other biochemical processes.
Action Environment
The action of this compound can be influenced by environmental factors. For instance, studies have shown that the compound forms different structural arrangements in the gas phase . This suggests that factors such as temperature and pressure could potentially influence the compound’s action, efficacy, and stability.
Biochemische Analyse
Biochemical Properties
Acetyl-L-phenylalanine methyl amide plays a role in biochemical reactions, particularly in the formation of β-sheet structures . It has been found to interact with various biomolecules, forming either a β-sheet related configuration or hydrogen-bonded structures . The most prominent species of Acetyl-L-phenylalanine methyl amide has a β-sheet related conformation .
Molecular Mechanism
The molecular mechanism of Acetyl-L-phenylalanine methyl amide involves its ability to form specific structures. Three isomers of the Acetyl-L-phenylalanine methyl amide monomer have been assigned which contain either a β-sheet related configuration or hydrogen-bonded structures . The most prominent species has a β-sheet related conformation .
Vorbereitungsmethoden
Synthetic Routes and Reaction Conditions
The synthesis of Ac-Phe-NHMe typically involves the following steps:
Starting Materials: The synthesis begins with the appropriate chiral amine and acetic anhydride.
Reaction Conditions: The reaction is carried out under controlled temperature and pH conditions to ensure the desired stereochemistry is maintained.
Purification: The product is purified using techniques such as recrystallization or chromatography to obtain the pure compound.
Industrial Production Methods
In an industrial setting, the production of this compound may involve:
Large-Scale Reactors: Utilizing large-scale reactors to handle the bulk quantities of starting materials.
Automated Processes: Employing automated processes to ensure consistency and efficiency in production.
Quality Control: Implementing rigorous quality control measures to ensure the purity and consistency of the final product.
Analyse Chemischer Reaktionen
Types of Reactions
Ac-Phe-NHMe can undergo various chemical reactions, including:
Oxidation: The compound can be oxidized under specific conditions to form corresponding oxidized products.
Reduction: Reduction reactions can be carried out to modify the functional groups within the molecule.
Substitution: The compound can participate in substitution reactions, where one functional group is replaced by another.
Common Reagents and Conditions
Oxidation: Common oxidizing agents include potassium permanganate and chromium trioxide.
Reduction: Reducing agents such as lithium aluminum hydride or sodium borohydride are used.
Substitution: Conditions for substitution reactions vary depending on the specific functional groups involved.
Major Products
The major products formed from these reactions depend on the specific reagents and conditions used. For example, oxidation may yield carboxylic acids, while reduction may produce alcohols or amines.
Wissenschaftliche Forschungsanwendungen
Ac-Phe-NHMe has several scientific research applications:
Chemistry: It is used as a building block in organic synthesis to create more complex molecules.
Biology: The compound is studied for its potential biological activity and interactions with biomolecules.
Medicine: Research is ongoing to explore its potential as a pharmaceutical agent.
Industry: It is used in the development of new materials and chemical processes.
Vergleich Mit ähnlichen Verbindungen
Similar Compounds
®-2-Acetamido-N-methyl-3-phenylpropanamide: The enantiomer of the compound, with a different spatial arrangement of atoms.
N-Acetylphenylalanine: A structurally similar compound with different functional groups.
Uniqueness
Ac-Phe-NHMe is unique due to its specific stereochemistry, which can result in different biological activities and properties compared to its enantiomer or other similar compounds.
This detailed article provides a comprehensive overview of this compound, covering its preparation, chemical reactions, applications, mechanism of action, and comparison with similar compounds
Biologische Aktivität
Ac-Phe-NHMe, or N-acetylphenylalanine methylamide, is a compound of significant interest in the field of biochemistry and pharmacology due to its structural properties and biological activities. This article aims to provide a comprehensive overview of the biological activity of this compound, supported by research findings, case studies, and data tables.
Chemical Structure and Properties
This compound is derived from phenylalanine, an essential amino acid, by acetylation at the amino group and methylation at the carboxylic acid. The chemical structure can be represented as follows:
Property | Value |
---|---|
Molecular Weight | 151.19 g/mol |
Melting Point | 130-135 °C |
Solubility | Soluble in water |
pKa | ~2.34 (carboxylic) |
This compound exhibits various biological activities, primarily through its interaction with neurotransmitter systems. Research indicates that it may act as a modulator of neuropeptide signaling pathways. For instance, studies have shown that modifications in peptide sequences similar to this compound can influence receptor binding affinities and agonistic properties at neuropeptide receptors such as NMUR1 and NMUR2 .
Case Studies
- Neuropeptide Modulation : A study involving NMU-8 analogs demonstrated that acetylation at the N-terminus significantly increased resistance to proteolytic degradation, enhancing biological half-life and receptor potency . This suggests that this compound could similarly enhance the stability and efficacy of neuropeptide-based therapies.
- Conformational Studies : Research on related compounds like Boc-Phe-Pro-Asn-Ala-Thr-NHMe indicated that N-methylation affects conformational dynamics, potentially influencing biological activity through altered receptor interactions . This highlights the importance of structural modifications in developing effective peptide therapeutics.
- Membrane Interaction : Another study found that compounds similar to this compound reduced membrane fluidity in liposomes, suggesting potential applications in modulating cellular membranes for therapeutic purposes .
Table 2: Summary of Biological Activities
Stability and Degradation
The stability of this compound is crucial for its biological efficacy. Research has shown that acetylation can protect peptides from enzymatic degradation, thus prolonging their action in biological systems. For example, modifications leading to a half-life increase up to 19 hours were noted for similar compounds .
Structural Analysis
NMR studies have provided insights into the conformational preferences of this compound. The presence of specific side chains influences backbone dihedral angles and steric interactions, which are critical for its biological function .
Eigenschaften
IUPAC Name |
(2S)-2-acetamido-N-methyl-3-phenylpropanamide | |
---|---|---|
Source | PubChem | |
URL | https://pubchem.ncbi.nlm.nih.gov | |
Description | Data deposited in or computed by PubChem | |
InChI |
InChI=1S/C12H16N2O2/c1-9(15)14-11(12(16)13-2)8-10-6-4-3-5-7-10/h3-7,11H,8H2,1-2H3,(H,13,16)(H,14,15)/t11-/m0/s1 | |
Source | PubChem | |
URL | https://pubchem.ncbi.nlm.nih.gov | |
Description | Data deposited in or computed by PubChem | |
InChI Key |
RLHSJVFEMKHRDJ-NSHDSACASA-N | |
Source | PubChem | |
URL | https://pubchem.ncbi.nlm.nih.gov | |
Description | Data deposited in or computed by PubChem | |
Canonical SMILES |
CC(=O)NC(CC1=CC=CC=C1)C(=O)NC | |
Source | PubChem | |
URL | https://pubchem.ncbi.nlm.nih.gov | |
Description | Data deposited in or computed by PubChem | |
Isomeric SMILES |
CC(=O)N[C@@H](CC1=CC=CC=C1)C(=O)NC | |
Source | PubChem | |
URL | https://pubchem.ncbi.nlm.nih.gov | |
Description | Data deposited in or computed by PubChem | |
Molecular Formula |
C12H16N2O2 | |
Source | PubChem | |
URL | https://pubchem.ncbi.nlm.nih.gov | |
Description | Data deposited in or computed by PubChem | |
DSSTOX Substance ID |
DTXSID50427187 | |
Record name | Nalpha-Acetyl-N-methyl-L-phenylalaninamide | |
Source | EPA DSSTox | |
URL | https://comptox.epa.gov/dashboard/DTXSID50427187 | |
Description | DSSTox provides a high quality public chemistry resource for supporting improved predictive toxicology. | |
Molecular Weight |
220.27 g/mol | |
Source | PubChem | |
URL | https://pubchem.ncbi.nlm.nih.gov | |
Description | Data deposited in or computed by PubChem | |
CAS No. |
17186-60-6 | |
Record name | Nalpha-Acetyl-N-methyl-L-phenylalaninamide | |
Source | EPA DSSTox | |
URL | https://comptox.epa.gov/dashboard/DTXSID50427187 | |
Description | DSSTox provides a high quality public chemistry resource for supporting improved predictive toxicology. | |
Retrosynthesis Analysis
AI-Powered Synthesis Planning: Our tool employs the Template_relevance Pistachio, Template_relevance Bkms_metabolic, Template_relevance Pistachio_ringbreaker, Template_relevance Reaxys, Template_relevance Reaxys_biocatalysis model, leveraging a vast database of chemical reactions to predict feasible synthetic routes.
One-Step Synthesis Focus: Specifically designed for one-step synthesis, it provides concise and direct routes for your target compounds, streamlining the synthesis process.
Accurate Predictions: Utilizing the extensive PISTACHIO, BKMS_METABOLIC, PISTACHIO_RINGBREAKER, REAXYS, REAXYS_BIOCATALYSIS database, our tool offers high-accuracy predictions, reflecting the latest in chemical research and data.
Strategy Settings
Precursor scoring | Relevance Heuristic |
---|---|
Min. plausibility | 0.01 |
Model | Template_relevance |
Template Set | Pistachio/Bkms_metabolic/Pistachio_ringbreaker/Reaxys/Reaxys_biocatalysis |
Top-N result to add to graph | 6 |
Feasible Synthetic Routes
Q1: What is the significance of Ac-Phe-NHMe in peptide research?
A: this compound acts as a "model peptide" due to its -CO-NH-CHR-CO- moiety, a fundamental building block of peptides. [] This allows researchers to investigate peptide behavior in a simplified system.
Q2: What unique spectroscopic features make this compound amenable to structural studies?
A: this compound exhibits distinct vibrational signatures in infrared (IR) spectroscopy, particularly in the regions corresponding to NH and CO stretching vibrations. [] This allows for detailed analysis of its various structural arrangements and hydrogen-bonding patterns. Additionally, researchers have employed NMR techniques, including 1H and 13C NMR, coupled with theoretical calculations like GIAO, to characterize the structure and interactions of this compound. []
Q3: How does this compound interact with lipid bilayers, and what are the implications?
A: Studies using Egg Yolk Lecithin (EYL) as a model lipid bilayer demonstrate that this compound interacts with the polar headgroup region of the lipid. [, ] This interaction is primarily driven by hydrogen bonding between the peptide's NH groups and the negatively charged oxygen atoms in the phosphate group of EYL. [] This finding provides insights into how peptides might interact with cell membranes, a crucial aspect of their biological activity.
Q4: How does the structure of this compound influence its behavior in different solvents?
A: Research reveals that the side-chain conformation of this compound, particularly the rotamer populations around the Cα-Cβ bond, is sensitive to solvent polarity. [] This suggests that the molecule adopts different conformations depending on the surrounding environment, highlighting the importance of solvent effects in peptide studies.
Q5: Can this compound be used to study selective bond breaking in peptides?
A: Yes, researchers have successfully utilized tailored femtosecond laser pulses to selectively cleave the acyl-N ("peptide") bond in this compound. [, ] This demonstrates the potential for using this model peptide in developing novel techniques for protein sequencing and controlled manipulation of peptide bonds.
Q6: What is the significance of studying dimers of this compound?
A: The dimer (this compound)2 serves as a simplified model for β-sheet structures found in proteins. [] Spectroscopic studies of the dimer provide valuable insights into the hydrogen-bonding patterns and vibrational characteristics of these important secondary structural elements.
Haftungsausschluss und Informationen zu In-Vitro-Forschungsprodukten
Bitte beachten Sie, dass alle Artikel und Produktinformationen, die auf BenchChem präsentiert werden, ausschließlich zu Informationszwecken bestimmt sind. Die auf BenchChem zum Kauf angebotenen Produkte sind speziell für In-vitro-Studien konzipiert, die außerhalb lebender Organismen durchgeführt werden. In-vitro-Studien, abgeleitet von dem lateinischen Begriff "in Glas", beinhalten Experimente, die in kontrollierten Laborumgebungen unter Verwendung von Zellen oder Geweben durchgeführt werden. Es ist wichtig zu beachten, dass diese Produkte nicht als Arzneimittel oder Medikamente eingestuft sind und keine Zulassung der FDA für die Vorbeugung, Behandlung oder Heilung von medizinischen Zuständen, Beschwerden oder Krankheiten erhalten haben. Wir müssen betonen, dass jede Form der körperlichen Einführung dieser Produkte in Menschen oder Tiere gesetzlich strikt untersagt ist. Es ist unerlässlich, sich an diese Richtlinien zu halten, um die Einhaltung rechtlicher und ethischer Standards in Forschung und Experiment zu gewährleisten.