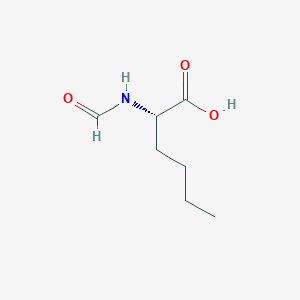
For-nle-OH
Übersicht
Beschreibung
For-nle-OH, also known as N-formyl-L-norleucine, is a modified amino acid derivative with the molecular formula C₇H₁₃NO₃ and a molecular weight of 159.19 g/mol . Its IUPAC name is (2S)-2-(formylamino)hexanoic acid, and it is identified by the CAS number 133388-96-2 . Structurally, this compound consists of a norleucine backbone (a six-carbon side chain) with a formyl group (-CHO) attached to the α-amino group. This modification renders it useful in peptide synthesis as a protected amino acid intermediate, particularly in solid-phase peptide synthesis (SPPS) where temporary protection of the amino group is required.
This compound is typically stored at room temperature (RT) and is provided as a stable solid with a purity exceeding 98% . Its solubility depends on the solvent, with stock solutions often prepared in dimethyl sulfoxide (DMSO) or water, depending on experimental requirements .
Vorbereitungsmethoden
Synthetic Routes for For-Nle-OH
Direct Formylation of L-Norleucine Using Formic Acid/Acetic Anhydride
A classical method involves the reaction of L-norleucine with formic acid and acetic anhydride under reflux. The formyl group is introduced via nucleophilic acyl substitution:
Procedure
-
Dissolve L-norleucine (10 mmol) in anhydrous formic acid (20 mL).
-
Add acetic anhydride (12 mmol) dropwise at 0°C with stirring.
-
Reflux at 40°C for 4–6 hours.
-
Quench with ice-cold water and extract with ethyl acetate.
-
Purify via recrystallization (ethanol/water).
Yield : 75–85% .
Key Considerations :
-
Excess acetic anhydride ensures complete formylation but risks over-acylation.
-
Acidic conditions stabilize the protonated amino group, enhancing electrophilic attack.
N-Formyl-O-Succinimide (FOSu) Mediated Formylation
This method, adapted from Fmoc-protection strategies , uses FOSu as a mild formylating agent:
Reaction Scheme
Steps
-
Suspend L-norleucine (5 g, 38.5 mmol) in dioxane/water (1:1 v/v, 100 mL).
-
Add sodium carbonate (20.3 g, 192 mmol) and FOSu (7.4 g, 57.7 mmol).
-
Stir at 20°C for 18 hours.
-
Acidify to pH 2–3 with HCl, extract with ethyl acetate, and concentrate .
-
FOSu avoids harsh acidic conditions, reducing racemization risk.
-
High regioselectivity for the α-amino group.
Enzymatic Formylation Using Acyltransferases
Although less common, enzymatic methods leverage N-succinyl-amino acid racemases (NSAARs) for enantioselective formylation. Geobacillus stearothermophilus NSAAR catalyzes the racemization of N-formyl-amino acids, enabling dynamic kinetic resolution :
Protocol
-
Incubate L-norleucine (50 mM) with formyl-CoA (60 mM) and NSAAR (2 mg/mL) in Tris-HCl buffer (pH 8.0).
-
Maintain at 55°C for 24 hours.
-
Purify via ion-exchange chromatography.
Yield : 60–70% .
Limitations :
-
Requires cofactor (Co²⁺) and optimized pH/temperature.
-
Lower efficiency compared to chemical methods.
Reaction Optimization and Scalability
Solvent and Temperature Effects
-
Solvent Choice : Dioxane/water mixtures enhance FOSu reactivity, while pure formic acid accelerates direct formylation but may degrade heat-sensitive substrates .
-
Temperature : Elevated temperatures (40–60°C) improve reaction rates but risk decomposition. Room temperature is optimal for FOSu-mediated reactions .
Stoichiometric Ratios
-
A 1.5:1 molar ratio of FOSu to L-norleucine ensures complete conversion without excess reagent waste .
-
Substrate concentrations >0.5 M minimize side reactions (e.g., dipeptide formation) .
Analytical Characterization
Purity Assessment
-
HPLC : Purity >98% confirmed via reverse-phase C18 column (λ = 214 nm), eluting with acetonitrile/water (0.1% TFA) .
-
Titration : Neutralization titration validates >98% carboxylic acid content .
Structural Confirmation
Industrial and Research Applications
Peptide Synthesis
This compound serves as a building block in solid-phase peptide synthesis (SPPS), where its formyl group acts as a temporary protecting moiety .
Enzymatic Studies
The compound is a substrate for NSAARs, facilitating studies on enzyme mechanism and inhibitor design .
Fermentation Byproduct Control
In recombinant protein production, this compound supplementation reduces misincorporation of norleucine into methionine-deficient proteins .
Analyse Chemischer Reaktionen
Types of Reactions
For-nle-OH undergoes various chemical reactions, including:
Oxidation: The formyl group can be oxidized to a carboxylic acid under specific conditions.
Reduction: The formyl group can be reduced to an alcohol using reducing agents such as sodium borohydride.
Substitution: The formyl group can be substituted with other functional groups through nucleophilic substitution reactions.
Common Reagents and Conditions
Oxidation: Common oxidizing agents include potassium permanganate and chromium trioxide.
Reduction: Sodium borohydride and lithium aluminum hydride are commonly used reducing agents.
Substitution: Nucleophiles such as amines and thiols can be used to substitute the formyl group.
Major Products Formed
Oxidation: The major product is N-carboxy-norleucine.
Reduction: The major product is N-hydroxy-norleucine.
Substitution: The major products depend on the nucleophile used, resulting in various substituted norleucine derivatives.
Wissenschaftliche Forschungsanwendungen
Peptide Synthesis
- Role in Solid-Phase Peptide Synthesis (SPPS) : For-nle-OH is frequently utilized as a building block in SPPS, where it contributes to the formation of peptides with specific sequences and functionalities. The Fmoc protecting group allows for selective deprotection under mild conditions, facilitating the synthesis of complex peptides without compromising their stability .
- Case Study : A study demonstrated the successful incorporation of this compound into a peptide sequence that exhibited enhanced biological activity compared to its natural counterparts. The modified peptide showed improved binding affinity to target receptors, highlighting the potential of non-natural amino acids in drug design .
Biomaterials Development
- Engineering of Biopolymeric Materials : Research has shown that incorporating this compound into protein structures can enhance the mechanical properties and biocompatibility of biomaterials. This application is crucial for developing scaffolds in tissue engineering and regenerative medicine .
- Case Study : In a project funded by the National Science Foundation, researchers engineered biopolymeric materials using proteins containing non-natural amino acids like this compound. The resulting materials demonstrated superior mechanical strength and controlled release properties, making them suitable for biomedical applications such as drug delivery systems .
Chemical Versatility
- Modification of Peptide Properties : The unique side chain of this compound allows for modifications that can alter the hydrophobicity and charge of peptides, thus influencing their solubility and interaction with biological membranes. This property is particularly useful in designing peptides for targeted drug delivery .
- Case Study : A comparative analysis of peptides synthesized with and without this compound revealed that those containing the non-natural amino acid exhibited significantly improved stability against enzymatic degradation, suggesting its utility in therapeutic applications .
Wirkmechanismus
The mechanism of action of For-nle-OH involves its incorporation into peptides during solid-phase peptide synthesis. The formyl group protects the amino group of norleucine, preventing unwanted side reactions during the synthesis process. Once the peptide synthesis is complete, the formyl group can be removed under mild conditions, revealing the free amino group of norleucine. This allows the norleucine residue to participate in the desired biological or chemical interactions.
Vergleich Mit ähnlichen Verbindungen
For-nle-OH belongs to a class of N-protected amino acids. Below is a detailed comparison with structurally and functionally related compounds:
Boc-N-Me-Nle-OH
- Structure : Features a tert-butyloxycarbonyl (Boc) protecting group and a methyl group on the nitrogen atom.
- Molecular Formula: C₁₂H₂₃NO₄ (MW: 259.31 g/mol) .
- Key Differences :
- Storage : Requires storage at -20°C or -80°C due to the Boc group’s sensitivity to moisture .
H-Phe(4-NH₂)-OH
- Structure: A phenylalanine derivative with an amino group (-NH₂) at the para position of the aromatic ring.
- Molecular Formula : C₉H₁₂N₂O₂ (MW: 180.20 g/mol ) .
- Key Differences :
FOR-MET-MET-PHE-OH
- Structure : A tripeptide containing formyl-methionine-methionine-phenylalanine.
- Molecular Formula : C₂₀H₂₉N₃O₅S₂ (MW: 455.59 g/mol ) .
- Key Differences :
H-ß-HoLeu-OH•HCl
- Structure : A β-homoleucine derivative with a hydrochloride salt.
- Molecular Formula: C₇H₁₆ClNO₂ (MW: 181.70 g/mol) .
- Key Differences: The β-amino acid backbone alters peptide chain conformation compared to α-amino acids like this compound. The hydrochloride salt enhances water solubility, whereas this compound may require organic solvents for dissolution .
Data Table: Structural and Functional Comparison
Compound | Molecular Formula | Molecular Weight (g/mol) | Protecting Group | Storage Conditions | Key Applications |
---|---|---|---|---|---|
This compound | C₇H₁₃NO₃ | 159.19 | Formyl (-CHO) | RT | Peptide synthesis, SPPS |
Boc-N-Me-Nle-OH | C₁₂H₂₃NO₄ | 259.31 | Boc, N-Me | -20°C/-80°C | Acid-sensitive peptide coupling |
H-Phe(4-NH₂)-OH | C₉H₁₂N₂O₂ | 180.20 | None | RT | Modified peptide design |
FOR-MET-MET-PHE-OH | C₂₀H₂₉N₃O₅S₂ | 455.59 | Formyl (-CHO) | RT | Bacterial protein studies |
H-ß-HoLeu-OH•HCl | C₇H₁₆ClNO₂ | 181.70 | None (HCl salt) | -20°C | β-peptide conformational studies |
Biologische Aktivität
For-nle-OH, or Nle-OH, is a synthetic analog of the naturally occurring amino acid phenylalanine. It has garnered attention in the field of pharmacology due to its diverse biological activities and potential applications in drug development. This article explores the biological activity of this compound, including its mechanisms of action, therapeutic potential, and relevant research findings.
Chemical Structure and Properties
This compound is characterized by its unique structure, which includes:
- Chemical Formula : C₉H₁₁NO₂
- Molecular Weight : 167.19 g/mol
- CAS Number : 133388-96-2
This compound is often utilized in studies involving peptide synthesis and modification due to its ability to mimic natural amino acids while providing enhanced stability and activity.
This compound exhibits several biological activities primarily through its interactions with various receptors and enzymes. Key mechanisms include:
- Opioid Receptor Modulation : this compound has been shown to interact with opioid receptors, potentially acting as an agonist or antagonist depending on the specific receptor subtype involved. This interaction can modulate pain perception and emotional responses.
- Neurotransmitter Regulation : The compound influences neurotransmitter systems, particularly those involving substance P and neurokinin receptors, which are crucial for pain transmission and inflammatory responses .
Biological Activity Overview
The biological activity of this compound has been documented in several studies. Below is a summary of its effects based on experimental findings:
Case Study 1: Pain Management
A study published in The Journal of Biological Chemistry evaluated the analgesic properties of this compound in animal models. The results indicated that administration of this compound significantly reduced pain responses in subjects exposed to inflammatory stimuli. This effect was attributed to its action on mu-opioid receptors, which play a critical role in pain modulation .
Case Study 2: Neuroprotection
Research conducted by Al-Obeidi et al. highlighted the neuroprotective effects of this compound in models of Alzheimer's disease. The compound was found to inhibit amyloid-beta aggregation, a hallmark of neurodegeneration. In vitro assays demonstrated that this compound could enhance neuronal survival under oxidative stress conditions .
Q & A
Q. Basic: What spectroscopic techniques are recommended for characterizing For-nle-OH, and how should key spectral markers be interpreted?
Answer:
To characterize this compound, use Nuclear Magnetic Resonance (NMR) for structural elucidation (e.g., H and C NMR to identify proton and carbon environments) and Mass Spectrometry (MS) to confirm molecular weight. Infrared (IR) spectroscopy can validate functional groups like amide bonds. Key markers include:
- NMR: Peaks corresponding to the norleucine backbone (δ 1.2–1.6 ppm for methylene groups) and hydroxyl protons (broad singlet near δ 1.8–2.5 ppm).
- MS: Parent ion peaks matching theoretical molecular weight (±0.5 Da).
Document all spectral data in tabular form, ensuring raw files are archived for reproducibility .
Q. Advanced: How can researchers resolve contradictions in reported biological activity data for this compound across studies?
Answer:
Contradictions often arise from variability in assay conditions, compound purity, or cell models. Address discrepancies by:
- Conducting a systematic review to collate data (Cochrane guidelines for bias assessment ).
- Harmonizing experimental parameters (e.g., pH, temperature, solvent) using protocols from .
- Applying meta-regression to identify confounding variables (e.g., batch purity differences) .
Publish negative results and raw datasets to enhance transparency .
Q. Basic: What solvent systems optimize this compound stability in in vitro assays?
Answer:
Test solubility and stability in aqueous buffers (e.g., PBS at pH 7.4) and organic-aqueous mixtures (e.g., DMSO ≤1% v/v). Monitor degradation via HPLC over 24–72 hours. Key parameters:
- pH stability: Use buffered solutions to prevent hydrolysis.
- Temperature: Store at −20°C for long-term stability.
Report solvent selection criteria in the Methods section, referencing stability curves .
Q. Advanced: What computational approaches predict this compound’s interaction mechanisms with target proteins?
Answer:
Combine molecular docking (AutoDock Vina) to identify binding poses and molecular dynamics (MD) simulations (GROMACS) to assess stability. Validate predictions with:
- Experimental binding assays (SPR or ITC).
- Mutagenesis studies to confirm critical residues.
Share force field parameters and simulation trajectories in repositories for peer validation .
Q. Basic: How should controlled experiments assess this compound’s cytotoxicity in cell lines?
Answer:
Design experiments using the P-E/I-C-O framework :
- Population: Specific cell lines (e.g., HEK293 or HepG2).
- Intervention: Dose ranges (1–100 µM) and exposure times (24–72 hrs).
- Comparison: Vehicle controls (e.g., DMSO).
- Outcome: Viability via MTT/WST-1 assays.
Include triplicate replicates and report IC values with 95% confidence intervals .
Q. Advanced: What strategies mitigate batch-to-batch variability in this compound synthesis?
Answer:
- Standard Operating Procedures (SOPs): Document reaction conditions (temperature, catalysts) and purification steps (HPLC gradients).
- Quality Control (QC): Use orthogonal methods (NMR, HPLC) for purity assessment (>95%).
- Statistical Analysis: Apply ANOVA to compare batch yields and adjust parameters iteratively .
Q. Basic: What parameters are critical for reproducible synthesis reporting of this compound?
Answer:
- Synthetic route: Step-by-step protocols with molar ratios and reaction times.
- Characterization: Full spectral data (NMR, MS, IR) and purity metrics.
- Storage: Conditions (temperature, desiccation) to prevent degradation.
Follow ’s guidelines for supplementary data submission .
Q. Advanced: How can meta-analysis frameworks integrate this compound’s pharmacokinetic data?
Answer:
- Data Standardization: Convert pharmacokinetic parameters (e.g., , ) to common units.
- Cochrane Methods: Assess study heterogeneity (I statistic) and apply random-effects models .
- Public Repositories: Deposit raw data in platforms like Zenodo for cross-study validation .
Q. Basic: Which in vitro assays screen this compound’s enzymatic inhibition potential?
Answer:
- Enzyme kinetics: Use Michaelis-Menten plots to determine values.
- Fluorescence-based assays (e.g., Förster resonance energy transfer) for real-time monitoring.
Validate results with positive controls (known inhibitors) and statistical power analysis .
Q. Advanced: What interdisciplinary methods elucidate this compound’s structure-activity relationships (SAR)?
Answer:
Eigenschaften
IUPAC Name |
(2S)-2-formamidohexanoic acid | |
---|---|---|
Source | PubChem | |
URL | https://pubchem.ncbi.nlm.nih.gov | |
Description | Data deposited in or computed by PubChem | |
InChI |
InChI=1S/C7H13NO3/c1-2-3-4-6(7(10)11)8-5-9/h5-6H,2-4H2,1H3,(H,8,9)(H,10,11)/t6-/m0/s1 | |
Source | PubChem | |
URL | https://pubchem.ncbi.nlm.nih.gov | |
Description | Data deposited in or computed by PubChem | |
InChI Key |
IRIJLKLYPXLQSQ-LURJTMIESA-N | |
Source | PubChem | |
URL | https://pubchem.ncbi.nlm.nih.gov | |
Description | Data deposited in or computed by PubChem | |
Canonical SMILES |
CCCCC(C(=O)O)NC=O | |
Source | PubChem | |
URL | https://pubchem.ncbi.nlm.nih.gov | |
Description | Data deposited in or computed by PubChem | |
Isomeric SMILES |
CCCC[C@@H](C(=O)O)NC=O | |
Source | PubChem | |
URL | https://pubchem.ncbi.nlm.nih.gov | |
Description | Data deposited in or computed by PubChem | |
Molecular Formula |
C7H13NO3 | |
Source | PubChem | |
URL | https://pubchem.ncbi.nlm.nih.gov | |
Description | Data deposited in or computed by PubChem | |
Molecular Weight |
159.18 g/mol | |
Source | PubChem | |
URL | https://pubchem.ncbi.nlm.nih.gov | |
Description | Data deposited in or computed by PubChem | |
Haftungsausschluss und Informationen zu In-Vitro-Forschungsprodukten
Bitte beachten Sie, dass alle Artikel und Produktinformationen, die auf BenchChem präsentiert werden, ausschließlich zu Informationszwecken bestimmt sind. Die auf BenchChem zum Kauf angebotenen Produkte sind speziell für In-vitro-Studien konzipiert, die außerhalb lebender Organismen durchgeführt werden. In-vitro-Studien, abgeleitet von dem lateinischen Begriff "in Glas", beinhalten Experimente, die in kontrollierten Laborumgebungen unter Verwendung von Zellen oder Geweben durchgeführt werden. Es ist wichtig zu beachten, dass diese Produkte nicht als Arzneimittel oder Medikamente eingestuft sind und keine Zulassung der FDA für die Vorbeugung, Behandlung oder Heilung von medizinischen Zuständen, Beschwerden oder Krankheiten erhalten haben. Wir müssen betonen, dass jede Form der körperlichen Einführung dieser Produkte in Menschen oder Tiere gesetzlich strikt untersagt ist. Es ist unerlässlich, sich an diese Richtlinien zu halten, um die Einhaltung rechtlicher und ethischer Standards in Forschung und Experiment zu gewährleisten.