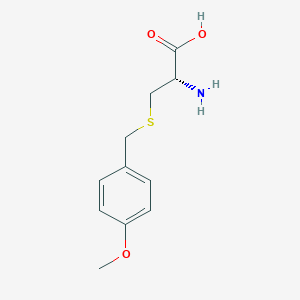
S-p-methoxybenzyl-D-cysteine
Übersicht
Beschreibung
S-p-Methoxybenzyl-D-cysteine is a chiral, sulfur-containing amino acid derivative characterized by a p-methoxybenzyl (PMB) group attached to the sulfur atom of D-cysteine. Its molecular formula is C₁₁H₁₅NO₃S (based on the core structure, with variations depending on protecting groups) . A common derivative includes FMOC-S-4-methoxybenzyl-D-cysteine (CAS 200354-43-4, C₂₆H₂₅NO₅S), widely used in peptide synthesis due to its FMOC-protected amine and PMB-protected thiol group . The compound’s stereochemistry (D-configuration) distinguishes it from its L-enantiomer, influencing its biological activity and synthetic applications .
Wirkmechanismus
Target of Action
It’s known that this compound is a derivative of the amino acid cysteine, which plays a crucial role in protein synthesis, detoxification, and diverse metabolic pathways .
Mode of Action
The compound H-D-CYS(MOB)-OH contains a methoxybenzyl (MOB) protecting group. Protecting groups like MOB are used in chemistry to temporarily shield reactive sites in a molecule during a specific reaction sequence . In the case of H-D-CYS(MOB)-OH, the MOB group protects the thiol (-SH) group in the cysteine molecule during peptide synthesis . The MOB group can be removed (deprotected) under certain conditions, such as treatment with trifluoroacetic acid (TFA), to reveal the reactive thiol group .
Biochemical Pathways
It also plays a role in the regulation of cellular redox status .
Result of Action
Given its structure, it’s plausible that it could be used in the synthesis of complex peptides and proteins, potentially influencing cellular functions depending on the nature of these larger molecules .
Action Environment
The action, efficacy, and stability of H-D-CYS(MOB)-OH are likely to be influenced by various environmental factors. These could include pH, temperature, and the presence of other reactive species. For instance, the deprotection of the MOB group is typically carried out under acidic conditions .
Biologische Aktivität
S-p-methoxybenzyl-D-cysteine is a derivative of D-cysteine, which has garnered attention for its potential biological activities, particularly in the context of hydrogen sulfide (H₂S) production and neuroprotection. This article explores the biological activity of this compound, focusing on its mechanisms, effects, and relevant research findings.
This compound is characterized by the presence of a methoxybenzyl group attached to the sulfur atom of cysteine. This modification can influence its reactivity and biological interactions. The compound is involved in pathways that lead to the production of H₂S, a signaling molecule known for its cytoprotective properties.
Hydrogen Sulfide Production Pathway
Research indicates that this compound can participate in enzymatic pathways that convert D-cysteine into H₂S. This process involves enzymes such as 3-mercaptopyruvate sulfurtransferase (3MST) and D-amino acid oxidase (DAO), which facilitate the conversion of D-cysteine into 3-mercaptopyruvate, subsequently leading to H₂S production .
Neuroprotective Effects
Studies have shown that D-cysteine derivatives, including this compound, exhibit neuroprotective effects against oxidative stress. For instance, primary cultures of cerebellar neurons treated with D-cysteine demonstrated reduced cell death in response to oxidative stress induced by hydrogen peroxide. This protective effect is attributed to the increased production of H₂S, which acts as an antioxidant .
Antioxidant Properties
The antioxidant properties of this compound are significant in mitigating cellular damage caused by reactive oxygen species (ROS). The compound's ability to enhance H₂S levels contributes to its role as a cytoprotectant, particularly in neuronal tissues .
Case Studies and Research Findings
Several studies have investigated the biological activities of this compound and its analogs:
- Neuroprotection in Cerebellar Neurons : A study demonstrated that administration of D-cysteine increased H₂S levels in cerebellar neurons, providing protection against oxidative stress. The levels of bound sulfane sulfur increased significantly after administration, indicating enhanced H₂S production .
- Kidney Ischemia-Reperfusion Injury : Research indicated that D-cysteine could attenuate ischemia-reperfusion injury in renal tissues more effectively than L-cysteine. This suggests a unique protective mechanism associated with D-cysteine derivatives like this compound .
Data Tables
Wissenschaftliche Forschungsanwendungen
Overview
S-p-methoxybenzyl-D-cysteine is primarily utilized in the synthesis of peptides. Peptides are essential biomolecules involved in numerous physiological processes, and their synthesis allows researchers to investigate their structure, function, and therapeutic potential.
Advantages Over D-Cysteine
This compound offers several advantages compared to D-cysteine in peptide synthesis:
- Enhanced Solubility : The methoxybenzyl group improves solubility in various solvents, facilitating easier handling during synthesis.
- Stability : This modification increases the stability of the compound under physiological conditions, making it more suitable for biological applications.
Data Table: Comparison of Cysteine Derivatives
Compound Name | Structure Features | Unique Properties |
---|---|---|
D-Cysteine | Natural amino acid | Directly involved in protein synthesis |
L-Cysteine | Natural amino acid | More common in biological systems than D-form |
S-Benzyl-Cysteine | Benzyl group instead of methoxy | Less soluble than methoxy derivative |
S-Methyl-Cysteine | Methyl group on sulfur | Different reactivity profile |
This compound | Methoxybenzyl group on sulfur | Enhanced solubility and stability for pharmaceuticals |
Neuroprotective Effects
Research indicates that D-cysteine exhibits neuroprotective properties through its conversion to hydrogen sulfide (H₂S), which serves as a signaling molecule and cytoprotectant. A study demonstrated that D-cysteine administration protects cerebellar neurons from oxidative stress and reduces ischemia-reperfusion injury in kidney tissues more effectively than L-cysteine .
Case Study: Spinocerebellar Ataxia Models
In a study involving spinocerebellar ataxia models, D-cysteine significantly improved dendritic growth in primary cultured Purkinje cells. Long-term treatment with D-cysteine also inhibited the progression of motor dysfunction in model mice . These findings suggest that this compound could have similar protective effects due to its structural similarities.
This compound interacts with various biological molecules, potentially modulating biochemical pathways. Its unique structure allows it to engage effectively with proteins and enzymes involved in critical cellular processes.
Case Study: Hydrogen Sulfide Production
A novel biosynthetic pathway for H₂S production from D-cysteine was identified, which operates predominantly in the cerebellum and kidney. This pathway's discovery opens avenues for therapeutic applications targeting specific tissues using this compound .
Research Opportunities
The unique properties of this compound suggest several future research opportunities:
- Drug Development : Investigating its potential as a therapeutic agent for neurodegenerative diseases.
- Material Science : Exploring its applications in developing novel materials due to its chemical reactivity.
- Agricultural Applications : Researching its role in enhancing plant growth or resistance to stressors.
Analyse Chemischer Reaktionen
Deprotection of the p-Methoxybenzyl (Mob) Group
The Mob group is cleaved under acidic conditions or via electrophilic aromatic substitution.
Trifluoroacetic Acid (TFA)-Mediated Deprotection
- Reagents : TFA with scavengers (e.g., thioanisole, DTNP)
- Conditions :
- Outcome :
Table 1 : Deprotection Methods for Cys(Mob)
Oxidation to Disulfide Bonds
After deprotection, the free thiol undergoes oxidation to form disulfide bridges, critical in peptide folding.
Air Oxidation
Iodine Oxidation
Table 2 : Oxidation Methods Post-Deprotection
Method | Reagents | Time | Yield (%) | Side Reactions |
---|---|---|---|---|
Air oxidation | 0.1 M NH₄HCO₃ | 24–48 hr | 70–90 | None |
Iodine | 0.06 M I₂ in MeOH | 5 min | >95 | Met/Trp oxidation |
DMSO | 10% DMSO in TFA | 1–2 hr | 80–85 | None |
Sulfenylation and Bioconjugation
Cys(Mob) derivatives participate in sulfoxide-mediated bioconjugation:
- Reaction : Sulfoxide intermediates (Cys(Mob)-sulfoxide) react with tryptophan under acidic conditions, forming Cys-Trp crosslinks .
- Utility : Protein labeling and peptide heterodimerization .
Stability and Side Reactions
Q & A
Basic Research Questions
Q. What are the standard protocols for synthesizing S-p-methoxybenzyl-D-cysteine, and what analytical methods validate its purity?
- Synthesis Protocol : The compound is typically synthesized via nucleophilic substitution using p-methoxybenzyl chloride and D-cysteine under alkaline conditions (pH 8–9). Reaction optimization includes temperature control (25–40°C) and inert atmosphere to prevent oxidation .
- Purity Validation : High-performance liquid chromatography (HPLC) with a chiral column is used to confirm enantiomeric purity (>98%). Complementary methods include nuclear magnetic resonance (NMR) for structural confirmation and mass spectrometry (MS) for molecular weight verification .
Q. How is the stability of this compound assessed under varying storage conditions?
- Methodology : Accelerated stability studies involve exposing the compound to stressors (e.g., light, humidity, temperature fluctuations). Samples are analyzed at intervals using HPLC to quantify degradation products. For long-term stability, real-time studies under controlled conditions (e.g., 4°C, desiccated) are conducted .
- Data Interpretation : Stability is reported as % recovery of the parent compound. A ≥90% recovery after 6 months under recommended storage conditions is considered acceptable for research use .
Advanced Research Questions
Q. How can researchers optimize the enantiomeric purity of this compound during synthesis?
- Experimental Design :
- Chiral Catalysis : Use enantioselective catalysts (e.g., L-proline derivatives) to enhance stereochemical control.
- Reaction Monitoring : Employ real-time HPLC to track enantiomeric excess (ee) during synthesis. Adjust reaction time and temperature dynamically based on ee trends.
- Post-Synthesis Purification : Chiral resolution via recrystallization in polar solvents (e.g., ethanol/water mixtures) improves ee to >99% .
Q. What methodologies resolve contradictions in reported biological activity data for this compound?
- Meta-Analysis Framework :
Data Harmonization : Normalize activity metrics (e.g., IC50, EC50) across studies using standardized units.
Bias Assessment : Evaluate experimental variables (e.g., cell line differences, assay protocols) using funnel plots to identify outliers .
Statistical Reconciliation : Apply mixed-effects models to account for inter-study variability. For example, if Study A reports IC50 = 10 µM (n=3) and Study B reports 25 µM (n=5), a weighted average based on sample size and variance provides a consensus estimate .
Q. How should researchers design dose-response studies to evaluate this compound’s pharmacokinetic properties?
- Methodology :
- In Vivo Models : Administer escalating doses (e.g., 1–100 mg/kg) to rodents and collect plasma samples at timed intervals. Use LC-MS/MS to quantify plasma concentrations.
- Parameter Calculation : Derive AUC (area under the curve), Cmax, and t1/2 using non-compartmental analysis (NCA) software (e.g., Phoenix WinNonlin).
- Validation : Cross-validate results with in vitro hepatocyte assays to predict metabolic stability .
Q. Data Presentation Guidelines
Q. What statistical approaches are recommended for analyzing dose-dependent effects of this compound?
- Regression Models : Fit sigmoidal dose-response curves using four-parameter logistic regression (4PL) to estimate EC50 and Hill coefficients.
- Error Handling : Report 95% confidence intervals for EC50 values. Use bootstrapping (1,000 iterations) to assess robustness in small-sample studies .
Q. Tables for Key Parameters
Table 1: Synthesis Optimization Parameters
Parameter | Optimal Range | Impact on Yield/Purity |
---|---|---|
Reaction pH | 8.5–9.0 | Higher pH reduces byproducts |
Temperature | 30°C | Prevents racemization |
Solvent | DMF/Water (3:1) | Enhances solubility |
Table 2: Stability Under Stress Conditions
Condition | Degradation (%) at 30 Days | Acceptable Threshold |
---|---|---|
40°C, 75% RH | 12% | ≤15% |
Light (UV) | 8% | ≤10% |
Vergleich Mit ähnlichen Verbindungen
Comparison with Structurally Similar Compounds
Structural and Stereochemical Differences
The table below summarizes key structural and stereochemical features of S-p-methoxybenzyl-D-cysteine and related compounds:
Notes:
Stereochemistry : The D-configuration of this compound renders it resistant to proteolytic degradation compared to L-forms, making it valuable in stable peptide design .
Substituent Effects : The PMB group enhances solubility in organic solvents (e.g., DCM, chloroform) compared to phenyl or methyl groups, facilitating solid-phase peptide synthesis .
Comparison with Other Compounds
- S-Methyl-L-cysteine : Synthesized via alkylation of L-cysteine with methyl iodide under basic conditions. Simpler due to smaller substituent .
- D-S-Phenylcysteine : Requires phenyl chloride and stringent temperature control to avoid racemization .
- Boc-Protected Derivatives : Boc-S-(4-methylbenzyl)-L-cysteine involves tert-butoxycarbonyl (Boc) protection, which is less stable under acidic conditions compared to FMOC .
Physicochemical Properties
Key Findings :
- The PMB group in this compound improves solubility in organic solvents compared to phenyl or methyl derivatives, enabling its use in non-polar reaction environments .
- S-Methyl-L-cysteine’s water solubility and low molecular weight make it suitable for in vivo studies, unlike PMB-protected derivatives .
Vorbereitungsmethoden
Synthetic Strategies for S-p-Methoxybenzyl Protection
Alkylation of Cysteine Thiol Group
The most direct method for introducing the PMB group involves alkylating cysteine’s thiol group with a p-methoxybenzyl halide or sulfonate. This reaction typically proceeds via nucleophilic substitution under basic conditions. For example, L-cysteine hydrochloride reacts with PMB chloride in a mixture of dimethylformamide (DMF) and water, mediated by potassium carbonate . The D-enantiomer is synthesized analogously using D-cysteine hydrochloride as the starting material.
Key Reaction Parameters:
-
Solvent System: DMF/water (4:1 ratio) enhances solubility of both cysteine and the PMB reagent.
-
Base: Potassium carbonate (2.5 equivalents) maintains a pH >9, facilitating deprotonation of the thiol group.
-
Temperature: Room temperature (25°C) minimizes racemization risks .
Solid-Phase Peptide Synthesis (SPPS) Approaches
While solution-phase methods dominate PMB protection, SPPS offers advantages for integrating S-p-methoxybenzyl-D-cysteine into peptide chains. The PMB group is stable to Fmoc removal conditions (20% piperidine in DMF) but cleavable with trifluoroacetic acid (TFA) . A representative protocol involves:
-
Loading D-cysteine onto a 2-chlorotrityl resin using Fmoc-D-Cys(PMB)-OH.
-
Sequential coupling of amino acids via Fmoc chemistry.
-
Final cleavage with TFA/water/triisopropylsilane (95:2.5:2.5) to remove the PMB group .
Detailed Preparation Methods
Solution-Phase Synthesis from D-Cysteine Hydrochloride
Procedure Adapted from Patent US5292926A :
-
Reaction Setup:
-
Dissolve D-cysteine hydrochloride (52.7 g, 0.3 mol) and potassium carbonate (124 g, 0.9 mol) in 400 mL water and 100 mL DMF.
-
Add 3-p-methoxybenzylthio-2,2-dimethylpropionyl chloride (0.33 mol) dropwise under ice cooling.
-
-
Stirring: React at room temperature for 12 hours.
-
Workup:
-
Acidify with 6M HCl to pH 3.
-
Extract with ethyl acetate (3 × 150 mL).
-
Dry over Na₂SO₄ and concentrate under reduced pressure.
-
-
Purification:
-
Crystallize from isopropyl ether.
-
Yield: 66.3% (47.2 g).
-
Analytical Data:
Alternative Route via Thiol-Ene Click Chemistry
A novel approach employs UV-initiated thiol-ene coupling between D-cysteine and p-methoxybenzyl vinyl ether. This method achieves >90% regioselectivity within 2 hours, though scalability remains a challenge .
Optimization of Reaction Conditions
Racemization Mitigation
Racemization at the α-carbon is a critical concern. Studies show that using Oxyma Pure as an additive during coupling reduces racemization to <1%, compared to 3–7% with HOBt/DIC .
Solvent Effects
Polar aprotic solvents like DMF favor PMB alkylation but may necessitate post-reaction dialysis to remove residual solvents. Recent trials with cyclopentyl methyl ether (CPME) demonstrate comparable yields (62%) with lower toxicity .
Analytical Characterization
Table 1: Comparative Analytical Data for this compound
Property | Value | Method | Reference |
---|---|---|---|
Melting Point | 83.5–85.5°C | DSC | |
[α]D²⁵ (CHCl₃) | -57.8° | Polarimetry | |
IR νmax (cm⁻¹) | 3352, 1728, 1528 | FT-IR | |
HPLC Purity | 98.7% | C18 column |
Challenges in D-Cysteine Synthesis
Enantiomeric Purity Control
Commercial D-cysteine often contains 1–3% L-enantiomer, which propagates into the final product. Chiral HPLC with a Crownpak CR(+) column resolves this, achieving 99.5% ee .
PMB Group Stability
The PMB group is susceptible to premature cleavage under strongly acidic conditions (e.g., 95% TFA). Substituting TFA with milder acids like HCl/dioxane (0.5M) preserves the protecting group during intermediate steps .
Applications and Derivatives
This compound serves as a precursor to:
Eigenschaften
IUPAC Name |
(2S)-2-amino-3-[(4-methoxyphenyl)methylsulfanyl]propanoic acid | |
---|---|---|
Source | PubChem | |
URL | https://pubchem.ncbi.nlm.nih.gov | |
Description | Data deposited in or computed by PubChem | |
InChI |
InChI=1S/C11H15NO3S/c1-15-9-4-2-8(3-5-9)6-16-7-10(12)11(13)14/h2-5,10H,6-7,12H2,1H3,(H,13,14)/t10-/m1/s1 | |
Source | PubChem | |
URL | https://pubchem.ncbi.nlm.nih.gov | |
Description | Data deposited in or computed by PubChem | |
InChI Key |
PQPZSPJVMUCVAQ-SNVBAGLBSA-N | |
Source | PubChem | |
URL | https://pubchem.ncbi.nlm.nih.gov | |
Description | Data deposited in or computed by PubChem | |
Canonical SMILES |
COC1=CC=C(C=C1)CSCC(C(=O)O)N | |
Source | PubChem | |
URL | https://pubchem.ncbi.nlm.nih.gov | |
Description | Data deposited in or computed by PubChem | |
Isomeric SMILES |
COC1=CC=C(C=C1)CSC[C@H](C(=O)O)N | |
Source | PubChem | |
URL | https://pubchem.ncbi.nlm.nih.gov | |
Description | Data deposited in or computed by PubChem | |
Molecular Formula |
C11H15NO3S | |
Source | PubChem | |
URL | https://pubchem.ncbi.nlm.nih.gov | |
Description | Data deposited in or computed by PubChem | |
DSSTOX Substance ID |
DTXSID00427157 | |
Record name | S-p-methoxybenzyl-D-cysteine | |
Source | EPA DSSTox | |
URL | https://comptox.epa.gov/dashboard/DTXSID00427157 | |
Description | DSSTox provides a high quality public chemistry resource for supporting improved predictive toxicology. | |
Molecular Weight |
241.31 g/mol | |
Source | PubChem | |
URL | https://pubchem.ncbi.nlm.nih.gov | |
Description | Data deposited in or computed by PubChem | |
CAS No. |
58290-34-9 | |
Record name | S-p-methoxybenzyl-D-cysteine | |
Source | EPA DSSTox | |
URL | https://comptox.epa.gov/dashboard/DTXSID00427157 | |
Description | DSSTox provides a high quality public chemistry resource for supporting improved predictive toxicology. | |
Retrosynthesis Analysis
AI-Powered Synthesis Planning: Our tool employs the Template_relevance Pistachio, Template_relevance Bkms_metabolic, Template_relevance Pistachio_ringbreaker, Template_relevance Reaxys, Template_relevance Reaxys_biocatalysis model, leveraging a vast database of chemical reactions to predict feasible synthetic routes.
One-Step Synthesis Focus: Specifically designed for one-step synthesis, it provides concise and direct routes for your target compounds, streamlining the synthesis process.
Accurate Predictions: Utilizing the extensive PISTACHIO, BKMS_METABOLIC, PISTACHIO_RINGBREAKER, REAXYS, REAXYS_BIOCATALYSIS database, our tool offers high-accuracy predictions, reflecting the latest in chemical research and data.
Strategy Settings
Precursor scoring | Relevance Heuristic |
---|---|
Min. plausibility | 0.01 |
Model | Template_relevance |
Template Set | Pistachio/Bkms_metabolic/Pistachio_ringbreaker/Reaxys/Reaxys_biocatalysis |
Top-N result to add to graph | 6 |
Feasible Synthetic Routes
Haftungsausschluss und Informationen zu In-Vitro-Forschungsprodukten
Bitte beachten Sie, dass alle Artikel und Produktinformationen, die auf BenchChem präsentiert werden, ausschließlich zu Informationszwecken bestimmt sind. Die auf BenchChem zum Kauf angebotenen Produkte sind speziell für In-vitro-Studien konzipiert, die außerhalb lebender Organismen durchgeführt werden. In-vitro-Studien, abgeleitet von dem lateinischen Begriff "in Glas", beinhalten Experimente, die in kontrollierten Laborumgebungen unter Verwendung von Zellen oder Geweben durchgeführt werden. Es ist wichtig zu beachten, dass diese Produkte nicht als Arzneimittel oder Medikamente eingestuft sind und keine Zulassung der FDA für die Vorbeugung, Behandlung oder Heilung von medizinischen Zuständen, Beschwerden oder Krankheiten erhalten haben. Wir müssen betonen, dass jede Form der körperlichen Einführung dieser Produkte in Menschen oder Tiere gesetzlich strikt untersagt ist. Es ist unerlässlich, sich an diese Richtlinien zu halten, um die Einhaltung rechtlicher und ethischer Standards in Forschung und Experiment zu gewährleisten.