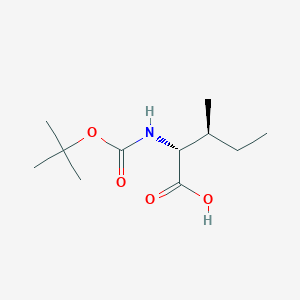
(Chloromethyl)polystyrene
Übersicht
Beschreibung
(Chloromethyl)polystyrene is a polymer that has been modified to include chloromethyl groups. This modification enhances its reactivity and makes it a valuable intermediate in the synthesis of various polymeric materials. The presence of chloromethyl groups allows for further functionalization, making it a versatile compound in both industrial and research applications.
Vorbereitungsmethoden
Synthetic Routes and Reaction Conditions: (Chloromethyl)polystyrene can be synthesized through several methods:
Direct Chloromethylation: This involves the reaction of polystyrene with chloromethyl ether in the presence of a catalyst such as ferric chloride.
Indirect Synthesis: This method uses p-chloromethyl styrene, styrene, and divinylbenzene as reactants.
Environmental-Friendly Method: A newer method involves the use of methylal, trioxymethylene, and thionyl chloride as reactants.
Industrial Production Methods: In industrial settings, the synthesis of this compound often involves the use of large-scale reactors and optimized reaction conditions to maximize yield and minimize costs. The choice of method depends on the desired properties of the final product and the specific application requirements.
Analyse Chemischer Reaktionen
(Chloromethyl)polystyrene undergoes various chemical reactions, including:
Substitution Reactions: The chloromethyl groups can be substituted with other functional groups through nucleophilic substitution reactions.
Cross-Linking Reactions: The chloromethyl groups can react with divinylbenzene to form cross-linked polymers, which are used in ion-exchange resins and other applications.
Oxidation and Reduction Reactions: While less common, this compound can undergo oxidation and reduction reactions under specific conditions.
Major Products:
Ion-Exchange Resins: Formed through cross-linking reactions.
Functionalized Polymers: Created through substitution reactions with various nucleophiles.
Wissenschaftliche Forschungsanwendungen
(Chloromethyl)polystyrene has a wide range of applications in scientific research:
Wirkmechanismus
The mechanism of action of (Chloromethyl)polystyrene primarily involves its reactivity due to the presence of chloromethyl groupsThis reactivity makes this compound a valuable intermediate in the synthesis of complex polymeric materials .
Vergleich Mit ähnlichen Verbindungen
Polystyrene: Lacks the chloromethyl groups, making it less reactive compared to (Chloromethyl)polystyrene.
Polydivinylbenzene: Often used in conjunction with this compound to create cross-linked polymers.
p-Chloromethyl Styrene: Used as a monomer in the indirect synthesis of this compound.
Uniqueness: this compound is unique due to its enhanced reactivity and versatility. The presence of chloromethyl groups allows for a wide range of chemical modifications, making it suitable for various applications in research and industry .
Biologische Aktivität
(Chloromethyl)polystyrene (CMS) is a functionalized polymer that has garnered attention due to its diverse applications in biological and chemical fields. This article explores the biological activity of CMS, focusing on its synthesis, properties, and applications in various biological contexts.
Synthesis of this compound
The synthesis of chloromethyl polystyrene involves chloromethylation of polystyrene using chloromethyl ether or chlorosulfonic acid. The process typically includes:
- Preparation : Mixing polystyrene with chloromethylating agents under controlled conditions.
- Reaction Conditions : The reaction is usually conducted at elevated temperatures (30-105 °C) to enhance the chloromethylation efficiency.
- Purification : Post-reaction, the product is purified through washing and extraction methods to remove unreacted materials and by-products.
A notable synthesis method reported a chlorine content exceeding 17% in the final product, indicating a high degree of functionalization .
Properties and Characterization
CMS exhibits several key properties that enhance its biological activity:
- Functional Groups : The presence of chloromethyl groups allows for further functionalization, enabling the attachment of various biological ligands or catalytic sites.
- Stability : CMS is chemically stable under various conditions, making it suitable for long-term applications in biological systems.
- Adsorption Capacity : The functionalized nature of CMS makes it an effective adsorbent for various biomolecules, including proteins and enzymes .
1. Ion Exchange Resins
CMS serves as a precursor for the synthesis of ion exchange resins (IERs), which are used in various biological applications:
- Catalysis : Sulfonated forms of CMS have been employed as solid acid catalysts in biochemical reactions, enhancing reaction rates and selectivity .
- Separation Techniques : IERs derived from CMS are utilized in the purification and separation of biomolecules, including nucleic acids and proteins.
2. Enzyme Immobilization
The ability to immobilize enzymes on CMS has significant implications for biocatalysis:
- Stability Enhancement : Enzymes immobilized on CMS exhibit increased thermal and operational stability compared to their free counterparts.
- Reusability : Immobilized enzymes can be reused multiple times, reducing costs in industrial applications .
3. Drug Delivery Systems
CMS has potential applications in drug delivery due to its functional properties:
- Controlled Release : The polymer can be engineered to release therapeutic agents in a controlled manner, enhancing treatment efficacy.
- Targeting Specific Tissues : Functionalization with targeting moieties allows for selective drug delivery to specific tissues or cells .
Case Study 1: Enzyme Immobilization on CMS
A study demonstrated the immobilization of lipase on chloromethylated polystyrene beads. The results showed that immobilized lipase retained over 80% of its activity after five cycles of use, highlighting the potential for CMS in enzyme-based applications.
Case Study 2: Synthesis of Solid Acid Catalysts
Research involving the synthesis of solid acid catalysts from CMS showed that these materials could effectively catalyze the hydrolysis of biomass-derived substrates, resulting in higher yields compared to traditional methods. This underscores the utility of CMS in sustainable bioprocessing .
Comparative Analysis Table
Property/Characteristic | Chloromethyl Polystyrene | Traditional Polystyrene |
---|---|---|
Chlorine Content | >17% | Not applicable |
Functionalization Potential | High | Low |
Stability | High | Moderate |
Application in Biocatalysis | Yes | Limited |
Use in Drug Delivery | Yes | No |
Eigenschaften
IUPAC Name |
(2R,3S)-3-methyl-2-[(2-methylpropan-2-yl)oxycarbonylamino]pentanoic acid | |
---|---|---|
Source | PubChem | |
URL | https://pubchem.ncbi.nlm.nih.gov | |
Description | Data deposited in or computed by PubChem | |
InChI |
InChI=1S/C11H21NO4/c1-6-7(2)8(9(13)14)12-10(15)16-11(3,4)5/h7-8H,6H2,1-5H3,(H,12,15)(H,13,14)/t7-,8+/m0/s1 | |
Source | PubChem | |
URL | https://pubchem.ncbi.nlm.nih.gov | |
Description | Data deposited in or computed by PubChem | |
InChI Key |
QJCNLJWUIOIMMF-JGVFFNPUSA-N | |
Source | PubChem | |
URL | https://pubchem.ncbi.nlm.nih.gov | |
Description | Data deposited in or computed by PubChem | |
Canonical SMILES |
CCC(C)C(C(=O)O)NC(=O)OC(C)(C)C | |
Source | PubChem | |
URL | https://pubchem.ncbi.nlm.nih.gov | |
Description | Data deposited in or computed by PubChem | |
Isomeric SMILES |
CC[C@H](C)[C@H](C(=O)O)NC(=O)OC(C)(C)C | |
Source | PubChem | |
URL | https://pubchem.ncbi.nlm.nih.gov | |
Description | Data deposited in or computed by PubChem | |
Molecular Formula |
C11H21NO4 | |
Source | PubChem | |
URL | https://pubchem.ncbi.nlm.nih.gov | |
Description | Data deposited in or computed by PubChem | |
DSSTOX Substance ID |
DTXSID301233370 | |
Record name | N-[(1,1-Dimethylethoxy)carbonyl]-D-alloisoleucine | |
Source | EPA DSSTox | |
URL | https://comptox.epa.gov/dashboard/DTXSID301233370 | |
Description | DSSTox provides a high quality public chemistry resource for supporting improved predictive toxicology. | |
Molecular Weight |
231.29 g/mol | |
Source | PubChem | |
URL | https://pubchem.ncbi.nlm.nih.gov | |
Description | Data deposited in or computed by PubChem | |
CAS No. |
55780-90-0, 13139-16-7 | |
Record name | N-[(1,1-Dimethylethoxy)carbonyl]-D-alloisoleucine | |
Source | CAS Common Chemistry | |
URL | https://commonchemistry.cas.org/detail?cas_rn=55780-90-0 | |
Description | CAS Common Chemistry is an open community resource for accessing chemical information. Nearly 500,000 chemical substances from CAS REGISTRY cover areas of community interest, including common and frequently regulated chemicals, and those relevant to high school and undergraduate chemistry classes. This chemical information, curated by our expert scientists, is provided in alignment with our mission as a division of the American Chemical Society. | |
Explanation | The data from CAS Common Chemistry is provided under a CC-BY-NC 4.0 license, unless otherwise stated. | |
Record name | L-Isoleucine, N-((1,1-dimethylethoxy)carbonyl)- | |
Source | ChemIDplus | |
URL | https://pubchem.ncbi.nlm.nih.gov/substance/?source=chemidplus&sourceid=0013139167 | |
Description | ChemIDplus is a free, web search system that provides access to the structure and nomenclature authority files used for the identification of chemical substances cited in National Library of Medicine (NLM) databases, including the TOXNET system. | |
Record name | N-[(1,1-Dimethylethoxy)carbonyl]-D-alloisoleucine | |
Source | EPA DSSTox | |
URL | https://comptox.epa.gov/dashboard/DTXSID301233370 | |
Description | DSSTox provides a high quality public chemistry resource for supporting improved predictive toxicology. | |
Retrosynthesis Analysis
AI-Powered Synthesis Planning: Our tool employs the Template_relevance Pistachio, Template_relevance Bkms_metabolic, Template_relevance Pistachio_ringbreaker, Template_relevance Reaxys, Template_relevance Reaxys_biocatalysis model, leveraging a vast database of chemical reactions to predict feasible synthetic routes.
One-Step Synthesis Focus: Specifically designed for one-step synthesis, it provides concise and direct routes for your target compounds, streamlining the synthesis process.
Accurate Predictions: Utilizing the extensive PISTACHIO, BKMS_METABOLIC, PISTACHIO_RINGBREAKER, REAXYS, REAXYS_BIOCATALYSIS database, our tool offers high-accuracy predictions, reflecting the latest in chemical research and data.
Strategy Settings
Precursor scoring | Relevance Heuristic |
---|---|
Min. plausibility | 0.01 |
Model | Template_relevance |
Template Set | Pistachio/Bkms_metabolic/Pistachio_ringbreaker/Reaxys/Reaxys_biocatalysis |
Top-N result to add to graph | 6 |
Feasible Synthetic Routes
Q1: How does CMPS interact with its target molecules?
A1: CMPS itself doesn't inherently target specific molecules. Its power lies in the reactivity of the chloromethyl group (-CH2Cl). This group readily undergoes nucleophilic substitution reactions, allowing for the attachment of various functional groups. These attached groups then dictate the interaction with target molecules. For instance, attaching a tertiary amine creates an anion exchange resin, as demonstrated by research using triethylamine (TEA) and methyldioctylamine (MDOA) to create resins for pertechnetate removal from groundwater [].
Q2: Can you give some examples of downstream effects after CMPS modification?
A2: The downstream effects depend entirely on the modification. Here are some examples from the provided research: * Selective metal ion adsorption: Modification with thiadiazole groups allows for selective palladium adsorption from wastewater []. * Catalytic activity: Introducing sulfonic acid groups creates a solid acid catalyst for cellulose hydrolysis [], []. * Controlled release: Grafting N-bromo-hydantoin enables the controlled release of oxidative bromine for water purification [].
Q3: What is the molecular formula and weight of CMPS?
A3: CMPS doesn't have a single, defined molecular formula or weight like small molecules. It's a polymer, meaning it's made of repeating units. The basic repeating unit of CMPS is styrene with a chloromethyl group attached: C8H7Cl. The 'n' denotes a variable number of repeating units, determining the molecular weight.
Q4: How is CMPS characterized spectroscopically?
A4: Common techniques include: * FTIR Spectroscopy: Identifies characteristic functional groups, like the C-Cl stretch in CMPS. Researchers used FTIR to confirm the incorporation of diaminopropane and β-cyclodextrin in a modified CMPS material []. * NMR Spectroscopy: Provides structural information about the polymer backbone and attached groups. For example, 1H and 13C NMR were used to characterize a new type of sulfur-doped polystyrene synthesized from CMPS []. * XPS (X-ray Photoelectron Spectroscopy): Analyzes elemental composition and chemical states of elements in the material. Researchers used XPS to study the adsorption mechanism of gold(I) thiosulfate complex on azole-functionalized CMPS microspheres [].
Q5: What are some applications where CMPS's material properties are advantageous?
A6: * Solid-phase synthesis: CMPS beads provide a robust and easily separable support for synthesizing peptides [], oligonucleotides [], and other molecules. * Chromatography: Modified CMPS resins serve as stationary phases for separating and purifying various compounds, such as α-amylase [] and p-nitrophenol []. * Adsorbents: Functionalized CMPS materials effectively remove heavy metal ions [], [], [] and organic pollutants [], [] from wastewater.
Q6: Can you provide examples of catalytic applications of modified CMPS?
A8: * Cellulose hydrolysis: Sulfonated CMPS effectively catalyzes the breakdown of cellulose into sugars like glucose and levulinic acid [], []. * Methanolysis of phosphatidylcholine: Quaternary ammonium functionalized CMPS catalyzes the conversion of natural phosphatidylcholine into glycerophosphocholine []. * Suzuki Coupling: N-heterocyclic carbene-palladium complexes supported on CMPS efficiently catalyze the formation of biaryl compounds from aryl bromides and arylboronic acids [].
Haftungsausschluss und Informationen zu In-Vitro-Forschungsprodukten
Bitte beachten Sie, dass alle Artikel und Produktinformationen, die auf BenchChem präsentiert werden, ausschließlich zu Informationszwecken bestimmt sind. Die auf BenchChem zum Kauf angebotenen Produkte sind speziell für In-vitro-Studien konzipiert, die außerhalb lebender Organismen durchgeführt werden. In-vitro-Studien, abgeleitet von dem lateinischen Begriff "in Glas", beinhalten Experimente, die in kontrollierten Laborumgebungen unter Verwendung von Zellen oder Geweben durchgeführt werden. Es ist wichtig zu beachten, dass diese Produkte nicht als Arzneimittel oder Medikamente eingestuft sind und keine Zulassung der FDA für die Vorbeugung, Behandlung oder Heilung von medizinischen Zuständen, Beschwerden oder Krankheiten erhalten haben. Wir müssen betonen, dass jede Form der körperlichen Einführung dieser Produkte in Menschen oder Tiere gesetzlich strikt untersagt ist. Es ist unerlässlich, sich an diese Richtlinien zu halten, um die Einhaltung rechtlicher und ethischer Standards in Forschung und Experiment zu gewährleisten.