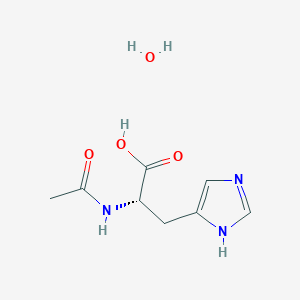
Acetyl histidine
Übersicht
Beschreibung
N-Acetyl-L-histidine (monohydrate) is a derivative of the amino acid histidine. It is a prominent biomolecule found in the brain, retina, and lens of poikilothermic vertebrates, such as fish, amphibians, and reptiles . This compound plays a significant role as an osmolyte, helping to maintain cellular osmotic balance.
Wirkmechanismus
Target of Action
Acetyl Histidine, also known as Ac-His-OH H2O, is a derivative of the essential amino acid L-histidine . The primary targets of this compound are likely to be similar to those of L-histidine, which plays unique roles in proton buffering, metal ion chelation, scavenging of reactive oxygen and nitrogen species, erythropoiesis, and the histaminergic system .
Mode of Action
For instance, it is involved in the synthesis of carnosine, histamine, and histidine-rich proteins . These molecules have specific functions that contribute to the overall physiological role of histidine.
Biochemical Pathways
Histidine, and by extension this compound, is involved in several biochemical pathways. It is a precursor for the synthesis of carnosine, a dipeptide that has antioxidant properties and plays a role in muscle function . Histidine is also converted into histamine, a neurotransmitter involved in immune response, digestion, and central nervous system function . Additionally, histidine participates in the synthesis of histidine-rich proteins and other derivatives .
Pharmacokinetics
It is known that n-acetylhistidine can be biosynthesized from l-histidine and acetyl-coa
Result of Action
The result of this compound’s action is likely to be similar to that of L-histidine, given that it is a derivative of this essential amino acid. L-histidine has unique roles in proton buffering, metal ion chelation, scavenging of reactive oxygen and nitrogen species, erythropoiesis, and the histaminergic system . These functions contribute to a wide range of physiological processes, including immune response, digestion, and central nervous system function.
Action Environment
The action of this compound, like that of L-histidine, can be influenced by various environmental factors. For instance, in the lens of poikilothermic vertebrates, N-acetyl-L-histidine exhibits a compartmentalized metabolism, suggesting that its action can be influenced by the specific cellular environment
Biochemische Analyse
Biochemical Properties
Acetyl histidine participates in numerous biochemical reactions. It interacts with various enzymes, proteins, and other biomolecules. For instance, it is involved in the synthesis of N-acetylhistidine through the action of histidine N-acetyltransferase . The nature of these interactions is complex and involves various biochemical pathways.
Cellular Effects
This compound influences various types of cells and cellular processes. It impacts cell function, including effects on cell signaling pathways, gene expression, and cellular metabolism
Molecular Mechanism
Acetyl-CoA plays a crucial role in many biochemical reactions, including the citric acid cycle and fatty acid synthesis .
Vorbereitungsmethoden
Synthetic Routes and Reaction Conditions: N-Acetyl-L-histidine (monohydrate) can be synthesized through the acetylation of L-histidine using acetic anhydride or acetyl chloride in the presence of a base such as pyridine. The reaction typically occurs at room temperature and is followed by purification steps such as recrystallization to obtain the monohydrate form .
Industrial Production Methods: Industrial production of N-Acetyl-L-histidine (monohydrate) involves large-scale acetylation processes using similar reagents and conditions as in laboratory synthesis. The process is optimized for higher yields and purity, often involving continuous flow reactors and advanced purification techniques .
Types of Reactions:
Oxidation: N-Acetyl-L-histidine (monohydrate) can undergo oxidation reactions, particularly at the imidazole ring, leading to the formation of various oxidation products.
Reduction: Reduction reactions are less common but can occur under specific conditions, such as the presence of strong reducing agents.
Substitution: The acetyl group can be substituted with other functional groups through nucleophilic substitution reactions.
Common Reagents and Conditions:
Oxidation: Common oxidizing agents include hydrogen peroxide and potassium permanganate.
Reduction: Reducing agents such as sodium borohydride can be used.
Substitution: Nucleophiles like amines or thiols can be used for substitution reactions.
Major Products:
Oxidation: Oxidized derivatives of N-Acetyl-L-histidine.
Reduction: Reduced forms of the compound.
Substitution: Substituted derivatives with various functional groups.
Wissenschaftliche Forschungsanwendungen
N-Acetyl-L-Histidin (Monohydrat) hat eine breite Palette von Anwendungen in der wissenschaftlichen Forschung:
Chemie: Wird als Modellverbindung zur Untersuchung von Acetylierungsreaktionen und dem Verhalten von Histidinderivaten verwendet.
Biologie: Untersucht auf seine Rolle als Osmolyt in zellulären Prozessen und seine Verteilung in verschiedenen Geweben.
Medizin: Erforscht auf potenzielle therapeutische Anwendungen, einschließlich seiner Rolle im Neuroschutz und als Biomarker für bestimmte Krankheiten.
5. Wirkmechanismus
N-Acetyl-L-Histidin (Monohydrat) entfaltet seine Wirkungen in erster Linie durch seine Rolle als Osmolyt. Es trägt zum osmotischen Gleichgewicht innerhalb der Zellen bei, indem es den Wassergehalt und die Ionenkonzentrationen reguliert. In der Linse poikilothermer Wirbeltiere wirkt es als molekulare Wasserpumpe, transportiert Wassermoleküle und verhindert die Bildung von Katarakten . Die Verbindung wird aus L-Histidin und Acetyl-Coenzym A synthetisiert und in Augenflüssigkeiten durch spezifische Acylasen hydrolysiert .
Ähnliche Verbindungen:
N-Acetyl-L-Aspartat: Eine weitere acetylierte Aminosäure, die im Gehirn und Auge vorkommt, insbesondere bei Vögeln und Säugetieren.
N-Acetyl-L-Cystein: Bekannt für seine antioxidativen Eigenschaften und Verwendung in medizinischen Behandlungen.
N-Acetyl-L-Methionin: Wird in der biochemischen Forschung und als Nahrungsergänzungsmittel verwendet.
Vergleich: N-Acetyl-L-Histidin (Monohydrat) ist in seiner spezifischen Rolle als Osmolyt in poikilothermen Wirbeltieren einzigartig. Im Gegensatz zu N-Acetyl-L-Aspartat, das bei homoiothermen Wirbeltieren häufiger vorkommt, findet sich N-Acetyl-L-Histidin hauptsächlich bei ektothermen Arten. Seine Funktion als molekulare Wasserpumpe in der Linse unterscheidet sich auch von den Rollen anderer acetylierter Aminosäuren .
Vergleich Mit ähnlichen Verbindungen
N-Acetyl-L-aspartate: Another acetylated amino acid found in the brain and eye, particularly in birds and mammals.
N-Acetyl-L-cysteine: Known for its antioxidant properties and use in medical treatments.
N-Acetyl-L-methionine: Used in biochemical research and as a dietary supplement.
Comparison: N-Acetyl-L-histidine (monohydrate) is unique in its specific role as an osmolyte in poikilothermic vertebrates. Unlike N-Acetyl-L-aspartate, which is more prevalent in homeothermic vertebrates, N-Acetyl-L-histidine is primarily found in ectothermic species. Its function as a molecular water pump in the lens is also distinct from the roles of other acetylated amino acids .
Biologische Aktivität
Acetyl histidine, specifically N-acetyl-L-histidine (NAH), is a derivative of the amino acid histidine that has garnered attention due to its potential biological activities. This article explores the biological activity of this compound, including its mechanisms of action, physiological roles, and implications in various studies.
N-acetyl-L-histidine is formed by the acetylation of the amino acid histidine. This modification can influence the compound's solubility, stability, and biological interactions. The acetyl group enhances the lipophilicity of histidine, potentially affecting its transport across biological membranes.
Biological Functions
-
Antioxidant Activity :
- NAH has been identified as a significant antioxidant in various biological systems. In studies involving fish, increased dietary histidine led to elevated levels of NAH in the lens, which correlated with reduced cataract formation, suggesting a protective role against oxidative stress in ocular tissues .
- Neurological Implications :
- Anti-inflammatory Effects :
The biological activity of this compound is attributed to several mechanisms:
-
Histone Acetylation :
Acetylation is a critical post-translational modification that regulates gene expression. Studies have shown that NAH can influence histone acetylation patterns, which may affect cellular responses to stress and inflammation . -
Interaction with Cellular Pathways :
NAH has been implicated in modulating pathways such as the Peroxisome proliferator-activated receptor (PPAR) signaling pathway. This modulation may influence lipid metabolism and energy homeostasis .
Table 1: Summary of Key Studies on this compound
Eigenschaften
IUPAC Name |
(2S)-2-acetamido-3-(1H-imidazol-5-yl)propanoic acid;hydrate | |
---|---|---|
Source | PubChem | |
URL | https://pubchem.ncbi.nlm.nih.gov | |
Description | Data deposited in or computed by PubChem | |
InChI |
InChI=1S/C8H11N3O3.H2O/c1-5(12)11-7(8(13)14)2-6-3-9-4-10-6;/h3-4,7H,2H2,1H3,(H,9,10)(H,11,12)(H,13,14);1H2/t7-;/m0./s1 | |
Source | PubChem | |
URL | https://pubchem.ncbi.nlm.nih.gov | |
Description | Data deposited in or computed by PubChem | |
InChI Key |
PSWSDQRXCOJSFC-FJXQXJEOSA-N | |
Source | PubChem | |
URL | https://pubchem.ncbi.nlm.nih.gov | |
Description | Data deposited in or computed by PubChem | |
Canonical SMILES |
CC(=O)NC(CC1=CN=CN1)C(=O)O.O | |
Source | PubChem | |
URL | https://pubchem.ncbi.nlm.nih.gov | |
Description | Data deposited in or computed by PubChem | |
Isomeric SMILES |
CC(=O)N[C@@H](CC1=CN=CN1)C(=O)O.O | |
Source | PubChem | |
URL | https://pubchem.ncbi.nlm.nih.gov | |
Description | Data deposited in or computed by PubChem | |
Molecular Formula |
C8H13N3O4 | |
Source | PubChem | |
URL | https://pubchem.ncbi.nlm.nih.gov | |
Description | Data deposited in or computed by PubChem | |
DSSTOX Substance ID |
DTXSID901021292 | |
Record name | N-Acetyl-L-histidine Monohydrate | |
Source | EPA DSSTox | |
URL | https://comptox.epa.gov/dashboard/DTXSID901021292 | |
Description | DSSTox provides a high quality public chemistry resource for supporting improved predictive toxicology. | |
Molecular Weight |
215.21 g/mol | |
Source | PubChem | |
URL | https://pubchem.ncbi.nlm.nih.gov | |
Description | Data deposited in or computed by PubChem | |
CAS No. |
39145-52-3 | |
Record name | Acetyl histidine | |
Source | ChemIDplus | |
URL | https://pubchem.ncbi.nlm.nih.gov/substance/?source=chemidplus&sourceid=0039145523 | |
Description | ChemIDplus is a free, web search system that provides access to the structure and nomenclature authority files used for the identification of chemical substances cited in National Library of Medicine (NLM) databases, including the TOXNET system. | |
Record name | N-Acetyl-L-histidine Monohydrate | |
Source | EPA DSSTox | |
URL | https://comptox.epa.gov/dashboard/DTXSID901021292 | |
Description | DSSTox provides a high quality public chemistry resource for supporting improved predictive toxicology. | |
Record name | N-ACETYLHISTIDINE MONOHYDRATE | |
Source | FDA Global Substance Registration System (GSRS) | |
URL | https://gsrs.ncats.nih.gov/ginas/app/beta/substances/PQ2TC3X11O | |
Description | The FDA Global Substance Registration System (GSRS) enables the efficient and accurate exchange of information on what substances are in regulated products. Instead of relying on names, which vary across regulatory domains, countries, and regions, the GSRS knowledge base makes it possible for substances to be defined by standardized, scientific descriptions. | |
Explanation | Unless otherwise noted, the contents of the FDA website (www.fda.gov), both text and graphics, are not copyrighted. They are in the public domain and may be republished, reprinted and otherwise used freely by anyone without the need to obtain permission from FDA. Credit to the U.S. Food and Drug Administration as the source is appreciated but not required. | |
Retrosynthesis Analysis
AI-Powered Synthesis Planning: Our tool employs the Template_relevance Pistachio, Template_relevance Bkms_metabolic, Template_relevance Pistachio_ringbreaker, Template_relevance Reaxys, Template_relevance Reaxys_biocatalysis model, leveraging a vast database of chemical reactions to predict feasible synthetic routes.
One-Step Synthesis Focus: Specifically designed for one-step synthesis, it provides concise and direct routes for your target compounds, streamlining the synthesis process.
Accurate Predictions: Utilizing the extensive PISTACHIO, BKMS_METABOLIC, PISTACHIO_RINGBREAKER, REAXYS, REAXYS_BIOCATALYSIS database, our tool offers high-accuracy predictions, reflecting the latest in chemical research and data.
Strategy Settings
Precursor scoring | Relevance Heuristic |
---|---|
Min. plausibility | 0.01 |
Model | Template_relevance |
Template Set | Pistachio/Bkms_metabolic/Pistachio_ringbreaker/Reaxys/Reaxys_biocatalysis |
Top-N result to add to graph | 6 |
Feasible Synthetic Routes
Haftungsausschluss und Informationen zu In-Vitro-Forschungsprodukten
Bitte beachten Sie, dass alle Artikel und Produktinformationen, die auf BenchChem präsentiert werden, ausschließlich zu Informationszwecken bestimmt sind. Die auf BenchChem zum Kauf angebotenen Produkte sind speziell für In-vitro-Studien konzipiert, die außerhalb lebender Organismen durchgeführt werden. In-vitro-Studien, abgeleitet von dem lateinischen Begriff "in Glas", beinhalten Experimente, die in kontrollierten Laborumgebungen unter Verwendung von Zellen oder Geweben durchgeführt werden. Es ist wichtig zu beachten, dass diese Produkte nicht als Arzneimittel oder Medikamente eingestuft sind und keine Zulassung der FDA für die Vorbeugung, Behandlung oder Heilung von medizinischen Zuständen, Beschwerden oder Krankheiten erhalten haben. Wir müssen betonen, dass jede Form der körperlichen Einführung dieser Produkte in Menschen oder Tiere gesetzlich strikt untersagt ist. Es ist unerlässlich, sich an diese Richtlinien zu halten, um die Einhaltung rechtlicher und ethischer Standards in Forschung und Experiment zu gewährleisten.