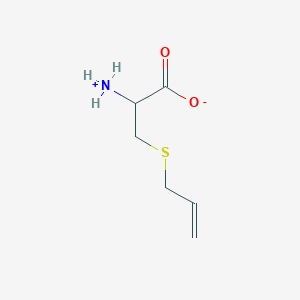
S-Allyl-L-Cystein
Übersicht
Beschreibung
S-allyl-L-cysteine is an organosulfur compound derived from the amino acid cysteine. It is primarily found in aged garlic and is known for its potential medicinal properties. The compound has the chemical formula HO₂CCH(NH₂)CH₂SCH₂CH=CH₂ and is significant biologically in its L-enantiomer form .
Wissenschaftliche Forschungsanwendungen
S-Allyl-L-Cystein hat eine breite Palette von Anwendungen in der wissenschaftlichen Forschung:
Chemie: Es wird als Vorläufer bei der Synthese anderer organischer Schwefelverbindungen verwendet.
Biologie: Die Verbindung wird auf ihre Rolle in zellulären Prozessen und Enzym-Interaktionen untersucht.
Medizin: this compound wird auf seine potenziellen Antikrebs-, antioxidativen und neuroprotektiven Eigenschaften untersucht.
Wirkmechanismus
This compound entfaltet seine Wirkungen durch verschiedene Mechanismen:
Antioxidative Aktivität: Es fängt freie Radikale ab und reduziert oxidativen Stress.
Enzymhemmung: Die Verbindung hemmt Enzyme wie Calpain, die an Zelltodwegen beteiligt sind.
Modulation der Genexpression: This compound beeinflusst die Expression von Genen, die mit antioxidativem Schutz und Apoptose zusammenhängen.
Wirkmechanismus
Target of Action
S-allyl-L-cysteine (SAC), also known as ®-3-(Allylthio)-2-aminopropanoic acid, is a sulfur-containing amino acid present in garlic . It exhibits a wide range of biological activities such as antioxidant, anti-inflammatory, and anticancer agent . The primary targets of SAC are the endoplasmic reticulum (ER) stress pathways and the enzyme calpain .
Mode of Action
SAC interacts with its targets primarily through two mechanisms. Firstly, it exerts significant protective effects against ER stress-induced neurotoxicity in neurons . Secondly, it directly suppresses calpain activity via binding to this enzyme’s Ca2+ binding domain .
Biochemical Pathways
SAC affects various biochemical pathways. It has been shown to ameliorate oxidative damage in a model of experimental stroke . ER stress and ER stress-induced cell death, which are involved in various neurological diseases, are also influenced by SAC . Furthermore, SAC and its derivatives have been shown to decrease the production of amyloid-β peptide in the brains of mice with D-galactose-induced aging .
Pharmacokinetics
SAC exhibits high oral bioavailability, indicating that it is well absorbed when administered orally . It undergoes N-acetylation and S-oxidation metabolism, and is extensively reabsorbed in the kidneys . This extensive renal reabsorption contributes to the long elimination half-life of SAC .
Result of Action
The molecular and cellular effects of SAC’s action are primarily neuroprotective. It protects neurons from ER stress-induced neurotoxicity . Moreover, it has been found to exert neuroprotective effects against dopaminergic neuron injury in a murine model of Parkinson’s disease .
Biochemische Analyse
Biochemical Properties
S-allyl-L-cysteine plays a crucial role in several biochemical reactions. It interacts with various enzymes, proteins, and other biomolecules, influencing their activity and function. One of the primary interactions of S-allyl-L-cysteine is with hydrogen sulfide-synthesizing enzymes such as 3-mercaptopyruvate sulfurtransferase, cystathionine γ-lyase, and cystathionine β-synthase . These interactions are essential for the modulation of hydrogen sulfide levels in cells, which in turn affects various physiological processes. Additionally, S-allyl-L-cysteine has been shown to inhibit prooxidant enzymes and chelate metals, contributing to its antioxidant properties .
Cellular Effects
S-allyl-L-cysteine exerts significant effects on various types of cells and cellular processes. In cancer cells, such as MCF-7 and MDA-MB-231 breast adenocarcinoma cell lines, S-allyl-L-cysteine has been shown to reduce cell proliferation and induce apoptosis . This compound also influences cell signaling pathways, including the downregulation of nuclear factor kappa B and the modulation of oxidative stress responses . Furthermore, S-allyl-L-cysteine affects gene expression by altering the expression of enzymes involved in hydrogen sulfide metabolism .
Molecular Mechanism
The molecular mechanism of S-allyl-L-cysteine involves several key interactions at the molecular level. S-allyl-L-cysteine binds to the Ca2±binding domain of calpain, an enzyme involved in neurotoxicity, thereby inhibiting its activity . This binding interaction is crucial for the neuroprotective effects of S-allyl-L-cysteine. Additionally, S-allyl-L-cysteine modulates the expression of genes involved in oxidative stress responses and inflammation, contributing to its antioxidant and anti-inflammatory properties .
Temporal Effects in Laboratory Settings
In laboratory settings, the effects of S-allyl-L-cysteine have been observed to change over time. Studies have shown that S-allyl-L-cysteine remains stable under various conditions, maintaining its biological activity . Long-term exposure to S-allyl-L-cysteine has been associated with sustained neuroprotective effects and the inhibition of oxidative stress-induced cell death . These findings suggest that S-allyl-L-cysteine can provide prolonged therapeutic benefits in in vitro and in vivo models.
Dosage Effects in Animal Models
The effects of S-allyl-L-cysteine vary with different dosages in animal models. At lower doses, S-allyl-L-cysteine has been shown to exert beneficial effects, such as reducing oxidative stress and inflammation . At higher doses, S-allyl-L-cysteine may exhibit toxic effects, including hepatotoxicity and nephrotoxicity . It is essential to determine the optimal dosage to maximize the therapeutic benefits while minimizing adverse effects.
Metabolic Pathways
S-allyl-L-cysteine is involved in several metabolic pathways, including the metabolism of hydrogen sulfide. It interacts with enzymes such as 3-mercaptopyruvate sulfurtransferase, cystathionine γ-lyase, and cystathionine β-synthase, which are involved in the endogenous formation of hydrogen sulfide from L-cysteine . These interactions are crucial for maintaining cellular redox balance and modulating various physiological processes.
Transport and Distribution
Within cells and tissues, S-allyl-L-cysteine is transported and distributed through specific transporters and binding proteins. The compound is known to interact with γ-glutamyl transpeptidase, which plays a role in its transport and distribution . This interaction facilitates the localization and accumulation of S-allyl-L-cysteine in specific cellular compartments, enhancing its biological activity.
Subcellular Localization
S-allyl-L-cysteine exhibits specific subcellular localization, which is essential for its activity and function. Studies have shown that S-allyl-L-cysteine localizes in the cytosol and interacts with various organelles, including the endoplasmic reticulum and mitochondria . This localization is mediated by targeting signals and post-translational modifications that direct S-allyl-L-cysteine to specific compartments, where it exerts its biological effects.
Vorbereitungsmethoden
Synthetische Routen und Reaktionsbedingungen
S-Allyl-L-Cystein kann durch verschiedene Methoden synthetisiert werden. Ein gängiger Ansatz beinhaltet die Reaktion von Cystein mit Allylbromid unter basischen Bedingungen. Die Reaktion verläuft typischerweise wie folgt:
- Cystein in Wasser lösen.
- Eine Base wie Natriumhydroxid zur Lösung hinzufügen.
- Allylbromid in das Gemisch einbringen.
- Das Reaktionsgemisch mehrere Stunden bei Raumtemperatur rühren.
- Das Produkt durch Kristallisation oder Chromatographie reinigen.
Industrielle Produktionsverfahren
Die industrielle Produktion von this compound erfolgt häufig durch Extraktion aus Knoblauch. Der Prozess umfasst:
- Frischen Knoblauch zerdrücken, um seine Säfte freizusetzen.
- Den Knoblauch altern lassen, währenddessen sich this compound auf natürliche Weise bildet.
- Die Verbindung mit Lösungsmitteln wie Ethanol oder Wasser extrahieren.
- Den Extrakt durch Filtration und Verdampfung reinigen .
Analyse Chemischer Reaktionen
Arten von Reaktionen
S-Allyl-L-Cystein durchläuft verschiedene Arten von chemischen Reaktionen, darunter:
Oxidation: Es kann oxidiert werden, um Sulfoxide und Sulfone zu bilden.
Reduktion: Die Verbindung kann reduziert werden, um Thiole zu bilden.
Substitution: this compound kann an nucleophilen Substitutionsreaktionen teilnehmen, bei denen die Allylgruppe durch andere funktionelle Gruppen ersetzt wird.
Häufige Reagenzien und Bedingungen
Oxidation: Häufige Oxidationsmittel sind Wasserstoffperoxid und Kaliumpermanganat.
Reduktion: Reduktionsmittel wie Natriumborhydrid und Lithiumaluminiumhydrid werden verwendet.
Substitution: Reagenzien wie Alkylhalogenide und Säuren werden unter basischen oder sauren Bedingungen eingesetzt.
Hauptprodukte, die gebildet werden
Oxidation: Sulfoxide und Sulfone.
Reduktion: Thiole.
Substitution: Verschiedene substituierte Cystein-Derivate.
Vergleich Mit ähnlichen Verbindungen
S-Allyl-L-Cystein ist im Vergleich zu anderen ähnlichen Verbindungen einzigartig aufgrund seiner spezifischen biologischen Aktivitäten und Stabilität. Ähnliche Verbindungen umfassen:
S-Allylmercaptocystein: Eine weitere organische Schwefelverbindung, die in Knoblauch vorkommt und für ihre krebshemmenden Eigenschaften bekannt ist.
γ-Glutamyl-S-Allylcystein: Ein Vorläufer von this compound, der ebenfalls in Knoblauch vorkommt.
S-Ethyl-L-Cystein: Ein Derivat mit ähnlichen neuroprotektiven Wirkungen, aber unterschiedlicher Potenz
This compound zeichnet sich durch seine gut dokumentierten gesundheitlichen Vorteile und sein Vorkommen in gealtertem Knoblauch aus, was es zu einer wertvollen Verbindung sowohl für die Forschung als auch für praktische Anwendungen macht.
Eigenschaften
IUPAC Name |
(2R)-2-amino-3-prop-2-enylsulfanylpropanoic acid | |
---|---|---|
Source | PubChem | |
URL | https://pubchem.ncbi.nlm.nih.gov | |
Description | Data deposited in or computed by PubChem | |
InChI |
InChI=1S/C6H11NO2S/c1-2-3-10-4-5(7)6(8)9/h2,5H,1,3-4,7H2,(H,8,9)/t5-/m0/s1 | |
Source | PubChem | |
URL | https://pubchem.ncbi.nlm.nih.gov | |
Description | Data deposited in or computed by PubChem | |
InChI Key |
ZFAHNWWNDFHPOH-YFKPBYRVSA-N | |
Source | PubChem | |
URL | https://pubchem.ncbi.nlm.nih.gov | |
Description | Data deposited in or computed by PubChem | |
Canonical SMILES |
C=CCSCC(C(=O)O)N | |
Source | PubChem | |
URL | https://pubchem.ncbi.nlm.nih.gov | |
Description | Data deposited in or computed by PubChem | |
Isomeric SMILES |
C=CCSC[C@@H](C(=O)O)N | |
Source | PubChem | |
URL | https://pubchem.ncbi.nlm.nih.gov | |
Description | Data deposited in or computed by PubChem | |
Molecular Formula |
C6H11NO2S | |
Source | PubChem | |
URL | https://pubchem.ncbi.nlm.nih.gov | |
Description | Data deposited in or computed by PubChem | |
DSSTOX Substance ID |
DTXSID20894862 | |
Record name | S-Allylcysteine | |
Source | EPA DSSTox | |
URL | https://comptox.epa.gov/dashboard/DTXSID20894862 | |
Description | DSSTox provides a high quality public chemistry resource for supporting improved predictive toxicology. | |
Molecular Weight |
161.22 g/mol | |
Source | PubChem | |
URL | https://pubchem.ncbi.nlm.nih.gov | |
Description | Data deposited in or computed by PubChem | |
CAS No. |
21593-77-1 | |
Record name | (+)-S-Allylcysteine | |
Source | CAS Common Chemistry | |
URL | https://commonchemistry.cas.org/detail?cas_rn=21593-77-1 | |
Description | CAS Common Chemistry is an open community resource for accessing chemical information. Nearly 500,000 chemical substances from CAS REGISTRY cover areas of community interest, including common and frequently regulated chemicals, and those relevant to high school and undergraduate chemistry classes. This chemical information, curated by our expert scientists, is provided in alignment with our mission as a division of the American Chemical Society. | |
Explanation | The data from CAS Common Chemistry is provided under a CC-BY-NC 4.0 license, unless otherwise stated. | |
Record name | S-Allylcysteine | |
Source | ChemIDplus | |
URL | https://pubchem.ncbi.nlm.nih.gov/substance/?source=chemidplus&sourceid=0021593771 | |
Description | ChemIDplus is a free, web search system that provides access to the structure and nomenclature authority files used for the identification of chemical substances cited in National Library of Medicine (NLM) databases, including the TOXNET system. | |
Record name | S-Allylcysteine | |
Source | EPA DSSTox | |
URL | https://comptox.epa.gov/dashboard/DTXSID20894862 | |
Description | DSSTox provides a high quality public chemistry resource for supporting improved predictive toxicology. | |
Record name | S-ALLYLCYSTEINE | |
Source | FDA Global Substance Registration System (GSRS) | |
URL | https://gsrs.ncats.nih.gov/ginas/app/beta/substances/81R3X99M15 | |
Description | The FDA Global Substance Registration System (GSRS) enables the efficient and accurate exchange of information on what substances are in regulated products. Instead of relying on names, which vary across regulatory domains, countries, and regions, the GSRS knowledge base makes it possible for substances to be defined by standardized, scientific descriptions. | |
Explanation | Unless otherwise noted, the contents of the FDA website (www.fda.gov), both text and graphics, are not copyrighted. They are in the public domain and may be republished, reprinted and otherwise used freely by anyone without the need to obtain permission from FDA. Credit to the U.S. Food and Drug Administration as the source is appreciated but not required. | |
Retrosynthesis Analysis
AI-Powered Synthesis Planning: Our tool employs the Template_relevance Pistachio, Template_relevance Bkms_metabolic, Template_relevance Pistachio_ringbreaker, Template_relevance Reaxys, Template_relevance Reaxys_biocatalysis model, leveraging a vast database of chemical reactions to predict feasible synthetic routes.
One-Step Synthesis Focus: Specifically designed for one-step synthesis, it provides concise and direct routes for your target compounds, streamlining the synthesis process.
Accurate Predictions: Utilizing the extensive PISTACHIO, BKMS_METABOLIC, PISTACHIO_RINGBREAKER, REAXYS, REAXYS_BIOCATALYSIS database, our tool offers high-accuracy predictions, reflecting the latest in chemical research and data.
Strategy Settings
Precursor scoring | Relevance Heuristic |
---|---|
Min. plausibility | 0.01 |
Model | Template_relevance |
Template Set | Pistachio/Bkms_metabolic/Pistachio_ringbreaker/Reaxys/Reaxys_biocatalysis |
Top-N result to add to graph | 6 |
Feasible Synthetic Routes
ANone: [] SAC can alleviate oxidative stress-induced injuries in chondrocytes, likely by downregulating genes involved in the Mitochondrial Inflammation Pathway (MIP). This includes genes like hypoxia-inducible factor 1α (Hif-1α), Xanthine Oxidase (XO), Caspase-9 (Casp-9), Caspase-3 (Casp-3), Interleukin 1 beta (IL-1β), and inducible nitric oxide synthase (iNOS). This downregulation leads to improved cell viability, morphology, and cell migration. Additionally, it decreases lactate dehydrogenase release, increases superoxide dismutase release, and enhances glycosaminoglycans retention. (Ref: S-ALLYL-L-CYSTEINE-INDUCED ANTI-INFLAMMATORY AND ANTI-APOPTOTIC EFFECTS IN CHONDROCYTES IS ASSOCIATED WITH SUPPRESSION OF THE MITOCHONDRIAL INFLAMMATION PATHWAY)
ANone: [] Yes, SAC has shown the ability to induce cell proliferation in specific contexts. For example, in primary cultures of adult rat hepatocytes, SAC stimulated time- and dose-dependent proliferation. This effect was linked to the autocrine secretion of Insulin-like Growth Factor 1 (IGF-1) triggered by SAC. The secreted IGF-1 subsequently activates the IGF-1 Receptor Tyrosine Kinase (RTK)/PI3K/MEK/ERK/mTOR pathway, ultimately leading to hepatocyte proliferation. (Ref: Signal transduction mechanism for S-allyl-L-cysteine-induced cell proliferation in primary cultures of adult rat hepatocytes)
ANone: [] The molecular formula of SAC is C6H11NO2S, and its molecular weight is 161.22 g/mol. (Ref: Isolation of (—)S-Allyl-L-cysteine from Garlic)
ANone: [] Yes, studies have utilized various spectroscopic techniques to characterize SAC. For instance, researchers employed optical rotatory dispersion (ORD) and circular dichroism (CD) to analyze the diastereomers of SAC S-oxides. These studies revealed the significant influence of the allyl group on the chiroptical properties of the sulfoxides. (Ref: Optical rotatory dispersion and circular dichroism of diastereomeric S-allyl-L-cysteine S-oxides)
ANone: [] The stability of SAC is influenced by cooking methods and conditions. While blanching and microwaving show minimal impact on SAC content, boiling and autoclaving lead to significant increases. Elevated temperatures and prolonged cooking times further amplify this increase in SAC content. (Ref: 마늘의 조리방법에 따른 S-Allyl-L-Cysteine 함량 변화)
ANone: [] While SAC itself is not an enzyme, it acts as a substrate for various enzymes. Notably, flavin-containing monooxygenases (FMOs) can catalyze the S-oxidation of SAC to its corresponding sulfoxide. The specific FMO isoform involved often depends on the structure of the cysteine conjugate. (Ref: Oxidation of cysteine S-conjugates by rabbit liver microsomes and cDNA-expressed flavin-containing mono-oxygenases: studies with S-(1,2-dichlorovinyl)-L-cysteine, S-(1,2,2-trichlorovinyl)-L-cysteine, S-allyl-L-cysteine, and S-benzyl-L-cysteine.)
ANone: [] SAC exhibits high oral bioavailability, exceeding 90%. It undergoes N-acetylation and S-oxidation during metabolism, and its elimination involves extensive renal reabsorption. The liver and kidneys play crucial roles in the elimination of SAC. (Ref: Metabolism, Excretion, and Pharmacokinetics of S-Allyl-l-Cysteine in Rats and Dogs)
ANone: [] SAC demonstrates neuroprotective properties, particularly in cerebral ischemic injury models. It has been shown to reduce infarct size following transient or global ischemic insults. This effect is attributed to its ability to scavenge peroxynitrite (ONOO−) and inhibit the activity of extracellular signal-regulated kinase (ERK). (Ref: S-Allyl-l-cysteine attenuates cerebral ischemic injury by scavenging peroxynitrite and inhibiting the activity of extracellular signal-regulated kinase)
ANone: [] Yes, research indicates that SAC can mitigate cadmium (Cd2+) stress in rice seedlings. It achieves this by influencing the expression of genes encoding cadmium transporter proteins. SAC treatment leads to reduced expression of OsNramp5 and OsHMA2, genes involved in Cd2+ uptake and transport, while increasing OsHMA3 expression, a gene associated with vacuolar sequestration of Cd2+. This altered gene expression profile contributes to reduced Cd2+ accumulation in the roots and shoots of rice seedlings. (Ref: [Mechanism of S-allyl-L-cysteine Alleviating Cadmium Stress in Seedling Roots and Buds of Rice Seedlings])
ANone: [] Yes, encapsulating SAC within chitosan nanoparticles (SC CS NPs) has been shown to improve its brain bioavailability. Intranasal administration of SC CS NPs resulted in significant enhancements in pharmacokinetic parameters, including area under the curve (AUC) and maximum concentration (Cmax), compared to intravenous and intranasal administration of free SAC. This improved bioavailability was correlated with enhanced neuroprotective effects in an ischemic stroke rat model, as evidenced by biochemical, neurobehavioral, and histopathological analyses. (Ref: Preparation of novel S-allyl cysteine chitosan based nanoparticles for use in ischemic brain treatment)
ANone: [] Yes, S-allyl-mercapturic acid (ALMA), a urinary metabolite of SAC, can be used as a biomarker to monitor garlic consumption. ALMA is formed through the metabolic pathway of γ-glutamyl-S-allyl-L-cysteine, a precursor found in garlic. Measurement of ALMA in urine samples using techniques like gas chromatography-mass spectrometry (GC-MS) can provide insights into garlic intake and compliance in dietary intervention studies. (Ref: Biomonitoring the intake of garlic via urinary excretion of allyl mercapturic acid)
ANone: [] A variety of analytical methods have been developed and validated for the accurate quantification of SAC in various matrices. These methods include:
Haftungsausschluss und Informationen zu In-Vitro-Forschungsprodukten
Bitte beachten Sie, dass alle Artikel und Produktinformationen, die auf BenchChem präsentiert werden, ausschließlich zu Informationszwecken bestimmt sind. Die auf BenchChem zum Kauf angebotenen Produkte sind speziell für In-vitro-Studien konzipiert, die außerhalb lebender Organismen durchgeführt werden. In-vitro-Studien, abgeleitet von dem lateinischen Begriff "in Glas", beinhalten Experimente, die in kontrollierten Laborumgebungen unter Verwendung von Zellen oder Geweben durchgeführt werden. Es ist wichtig zu beachten, dass diese Produkte nicht als Arzneimittel oder Medikamente eingestuft sind und keine Zulassung der FDA für die Vorbeugung, Behandlung oder Heilung von medizinischen Zuständen, Beschwerden oder Krankheiten erhalten haben. Wir müssen betonen, dass jede Form der körperlichen Einführung dieser Produkte in Menschen oder Tiere gesetzlich strikt untersagt ist. Es ist unerlässlich, sich an diese Richtlinien zu halten, um die Einhaltung rechtlicher und ethischer Standards in Forschung und Experiment zu gewährleisten.