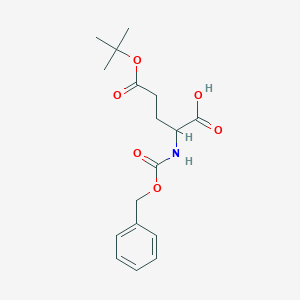
z-d-Glu(otbu)-oh
Übersicht
Beschreibung
Z-D-Glutamic Acid Tert-Butyl Ester: (Z-D-Glu(otbu)-oh) is a derivative of glutamic acid, an important amino acid in biochemistry. This compound is often used in peptide synthesis as a protecting group for the carboxyl group of glutamic acid. The presence of the tert-butyl ester group helps to prevent unwanted reactions during the synthesis process, making it a valuable tool in organic chemistry and biochemistry.
Vorbereitungsmethoden
Synthetic Routes and Reaction Conditions: The synthesis of Z-D-Glu(otbu)-oh typically involves the esterification of glutamic acid with tert-butyl alcohol in the presence of a suitable catalyst. The process can be summarized as follows:
Starting Materials: Glutamic acid, tert-butyl alcohol, and a catalyst such as dicyclohexylcarbodiimide (DCC) or 1-ethyl-3-(3-dimethylaminopropyl)carbodiimide (EDC).
Reaction Conditions: The reaction is carried out in an appropriate solvent, such as dichloromethane or dimethylformamide, at room temperature.
Procedure: Glutamic acid is dissolved in the solvent, and tert-butyl alcohol is added. The catalyst is then introduced to the mixture, and the reaction is allowed to proceed for several hours. The product is isolated by filtration or extraction and purified by recrystallization or chromatography.
Industrial Production Methods: Industrial production of this compound follows similar principles but on a larger scale. The process involves the use of industrial-grade solvents and catalysts, and the reaction conditions are optimized for maximum yield and purity. The product is typically purified using large-scale chromatography or crystallization techniques.
Analyse Chemischer Reaktionen
Types of Reactions: Z-D-Glu(otbu)-oh undergoes various chemical reactions, including:
Hydrolysis: The tert-butyl ester group can be hydrolyzed under acidic or basic conditions to yield the free carboxylic acid.
Substitution: The compound can participate in nucleophilic substitution reactions, where the ester group is replaced by other functional groups.
Oxidation and Reduction: While less common, the compound can undergo oxidation and reduction reactions under specific conditions.
Common Reagents and Conditions:
Hydrolysis: Acidic conditions (e.g., hydrochloric acid) or basic conditions (e.g., sodium hydroxide).
Substitution: Nucleophiles such as amines or alcohols in the presence of a suitable catalyst.
Oxidation and Reduction: Oxidizing agents like potassium permanganate or reducing agents like lithium aluminum hydride.
Major Products:
Hydrolysis: Free glutamic acid.
Substitution: Various substituted derivatives of glutamic acid.
Oxidation and Reduction: Oxidized or reduced forms of the compound, depending on the specific reaction conditions.
Wissenschaftliche Forschungsanwendungen
Chemistry: Z-D-Glu(otbu)-oh is widely used in peptide synthesis as a protecting group for the carboxyl group of glutamic acid. This allows for the selective deprotection and coupling of amino acids, facilitating the synthesis of complex peptides and proteins.
Biology: In biological research, this compound is used to study the role of glutamic acid in various biochemical pathways. It serves as a model compound for investigating enzyme-substrate interactions and protein folding mechanisms.
Medicine: The compound is used in the development of peptide-based drugs and therapeutic agents. Its ability to protect the carboxyl group of glutamic acid makes it valuable in the synthesis of bioactive peptides with potential medical applications.
Industry: this compound is employed in the production of specialty chemicals and materials. Its use in peptide synthesis extends to the manufacture of pharmaceuticals, agrochemicals, and other high-value products.
Wirkmechanismus
Mechanism: The primary function of Z-D-Glu(otbu)-oh is to protect the carboxyl group of glutamic acid during peptide synthesis. The tert-butyl ester group prevents unwanted reactions by blocking the carboxyl group, allowing for selective deprotection and coupling of amino acids.
Molecular Targets and Pathways: The compound interacts with various enzymes and proteins involved in peptide synthesis. It is recognized by peptide synthesis machinery, facilitating the incorporation of glutamic acid into peptides and proteins. The tert-butyl ester group is eventually removed under specific conditions, yielding the free carboxyl group of glutamic acid.
Vergleich Mit ähnlichen Verbindungen
Z-L-Glu(otbu)-oh: The L-isomer of Z-D-Glu(otbu)-oh, used in similar applications but with different stereochemistry.
Cbz-Glu(otbu)-oh: Another protecting group for glutamic acid, with a different protecting group for the amino group.
Fmoc-Glu(otbu)-oh: A compound used in solid-phase peptide synthesis with a different protecting group for the amino group.
Uniqueness: this compound is unique due to its specific stereochemistry (D-isomer) and the presence of the tert-butyl ester group. This combination provides distinct advantages in peptide synthesis, allowing for selective protection and deprotection of the carboxyl group. The D-isomer also offers different biological properties compared to the L-isomer, making it valuable in specific research and industrial applications.
Biologische Aktivität
Z-D-Glu(otbu)-oh, also known as Z-D-glutamic acid α-t-butyl ester, is a derivative of D-glutamic acid that plays a significant role in biochemical research and pharmaceutical applications. This compound is characterized by the presence of a benzyloxycarbonyl (Z) protecting group on the amino group and a t-butyl ester protecting the carboxylic acid group. Its unique structure allows it to be utilized effectively in peptide synthesis and other biological applications.
This compound functions primarily as a protected amino acid derivative in peptide synthesis. The benzyloxycarbonyl group protects the amino group from unwanted reactions during synthesis, while the t-butyl ester can be selectively removed to incorporate the amino acid into peptide chains. This dual protection strategy enhances the stability and reactivity of the compound, making it suitable for various biochemical applications.
Applications in Research
- Peptide Synthesis : this compound is widely used as a building block in the synthesis of peptides. Its stability under various conditions allows researchers to create complex peptide structures essential for studying protein function and interactions .
- Drug Development : The compound has been employed in the development of peptide-based drugs targeting diseases such as cancer and infectious diseases. Its ability to facilitate the formation of bioactive peptides makes it a valuable reagent in medicinal chemistry .
- Structural Biology : In structural biology, this compound is utilized to study protein structures and functions. By incorporating this compound into peptides, researchers can investigate how specific amino acid sequences influence protein folding and stability .
Case Study 1: Peptide Cyclization
A study explored the use of this compound in copper(I)-mediated azide-alkyne cycloaddition (CuAAC) reactions for peptide cyclization. The results indicated that peptides containing this compound could undergo efficient cyclodimerization, enhancing their biological activity through structural modifications .
Case Study 2: Cancer Therapeutics
Research has highlighted the potential of peptides synthesized using this compound in targeting cancer cells. For instance, peptides developed from this compound were shown to inhibit cell proliferation in colon cancer models, suggesting its utility in creating therapeutics that can selectively target cancerous tissues .
Comparative Analysis with Similar Compounds
To understand the unique properties of this compound, it is beneficial to compare it with other similar compounds used in peptide synthesis:
Compound | Key Features | Applications |
---|---|---|
N-Cbz-D-glutamic acid | Cbz protecting group; stable under acidic conditions | Peptide synthesis |
Boc-Glu(otbu)-oh | Boc protecting group; less steric hindrance | Drug development |
Fmoc-Glu(otbu)-oh | Fmoc protecting group; ideal for solid-phase synthesis | High-throughput screening |
This compound stands out due to its combination of stability and reactivity, making it particularly effective for synthesizing complex peptides that require precise control over reaction conditions.
Eigenschaften
IUPAC Name |
(2R)-5-[(2-methylpropan-2-yl)oxy]-5-oxo-2-(phenylmethoxycarbonylamino)pentanoic acid | |
---|---|---|
Source | PubChem | |
URL | https://pubchem.ncbi.nlm.nih.gov | |
Description | Data deposited in or computed by PubChem | |
InChI |
InChI=1S/C17H23NO6/c1-17(2,3)24-14(19)10-9-13(15(20)21)18-16(22)23-11-12-7-5-4-6-8-12/h4-8,13H,9-11H2,1-3H3,(H,18,22)(H,20,21)/t13-/m1/s1 | |
Source | PubChem | |
URL | https://pubchem.ncbi.nlm.nih.gov | |
Description | Data deposited in or computed by PubChem | |
InChI Key |
GLMODRZPPBZPPB-CYBMUJFWSA-N | |
Source | PubChem | |
URL | https://pubchem.ncbi.nlm.nih.gov | |
Description | Data deposited in or computed by PubChem | |
Canonical SMILES |
CC(C)(C)OC(=O)CCC(C(=O)O)NC(=O)OCC1=CC=CC=C1 | |
Source | PubChem | |
URL | https://pubchem.ncbi.nlm.nih.gov | |
Description | Data deposited in or computed by PubChem | |
Isomeric SMILES |
CC(C)(C)OC(=O)CC[C@H](C(=O)O)NC(=O)OCC1=CC=CC=C1 | |
Source | PubChem | |
URL | https://pubchem.ncbi.nlm.nih.gov | |
Description | Data deposited in or computed by PubChem | |
Molecular Formula |
C17H23NO6 | |
Source | PubChem | |
URL | https://pubchem.ncbi.nlm.nih.gov | |
Description | Data deposited in or computed by PubChem | |
Molecular Weight |
337.4 g/mol | |
Source | PubChem | |
URL | https://pubchem.ncbi.nlm.nih.gov | |
Description | Data deposited in or computed by PubChem | |
Retrosynthesis Analysis
AI-Powered Synthesis Planning: Our tool employs the Template_relevance Pistachio, Template_relevance Bkms_metabolic, Template_relevance Pistachio_ringbreaker, Template_relevance Reaxys, Template_relevance Reaxys_biocatalysis model, leveraging a vast database of chemical reactions to predict feasible synthetic routes.
One-Step Synthesis Focus: Specifically designed for one-step synthesis, it provides concise and direct routes for your target compounds, streamlining the synthesis process.
Accurate Predictions: Utilizing the extensive PISTACHIO, BKMS_METABOLIC, PISTACHIO_RINGBREAKER, REAXYS, REAXYS_BIOCATALYSIS database, our tool offers high-accuracy predictions, reflecting the latest in chemical research and data.
Strategy Settings
Precursor scoring | Relevance Heuristic |
---|---|
Min. plausibility | 0.01 |
Model | Template_relevance |
Template Set | Pistachio/Bkms_metabolic/Pistachio_ringbreaker/Reaxys/Reaxys_biocatalysis |
Top-N result to add to graph | 6 |
Feasible Synthetic Routes
Haftungsausschluss und Informationen zu In-Vitro-Forschungsprodukten
Bitte beachten Sie, dass alle Artikel und Produktinformationen, die auf BenchChem präsentiert werden, ausschließlich zu Informationszwecken bestimmt sind. Die auf BenchChem zum Kauf angebotenen Produkte sind speziell für In-vitro-Studien konzipiert, die außerhalb lebender Organismen durchgeführt werden. In-vitro-Studien, abgeleitet von dem lateinischen Begriff "in Glas", beinhalten Experimente, die in kontrollierten Laborumgebungen unter Verwendung von Zellen oder Geweben durchgeführt werden. Es ist wichtig zu beachten, dass diese Produkte nicht als Arzneimittel oder Medikamente eingestuft sind und keine Zulassung der FDA für die Vorbeugung, Behandlung oder Heilung von medizinischen Zuständen, Beschwerden oder Krankheiten erhalten haben. Wir müssen betonen, dass jede Form der körperlichen Einführung dieser Produkte in Menschen oder Tiere gesetzlich strikt untersagt ist. Es ist unerlässlich, sich an diese Richtlinien zu halten, um die Einhaltung rechtlicher und ethischer Standards in Forschung und Experiment zu gewährleisten.