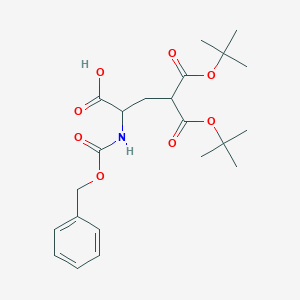
Z-DL-Gla(OtBu)2-OH
Übersicht
Beschreibung
Z-DL-Gla(OtBu)₂-OH is a protected derivative of γ-carboxyglutamic acid (Gla), a non-proteinogenic amino acid critical in biological processes such as blood coagulation and bone metabolism. The compound features a benzyloxycarbonyl (Z) group protecting the α-amino group and two tert-butoxy (OtBu) groups shielding the γ-carboxylic acid moieties. This protection strategy enhances stability during solid-phase peptide synthesis (SPPS) and prevents undesired side reactions. Z-DL-Gla(OtBu)₂-OH is widely used in medicinal chemistry to synthesize peptides requiring Gla residues, such as vitamin K-dependent proteins .
Vorbereitungsmethoden
The synthesis of Z-DL-Gla(OtBu)₂-OH typically involves multi-step protection-deprotection strategies to preserve the integrity of reactive functional groups. The most widely cited method, reported by Somlai et al. (1997), employs a two-step process starting from di-tert-butyl malonate .
Step 1: Alkylation and Esterification
Reagents : Sodium metal, tetrahydrofuran (THF)
Conditions : Ambient temperature, anhydrous atmosphere
Mechanism : Sodium acts as a base, deprotonating the malonate to form a nucleophilic enolate. This intermediate undergoes alkylation with a suitable electrophile (e.g., bromoacetate derivatives) to construct the glutamic acid backbone. The tert-butyl esters remain intact under these conditions, ensuring selective protection of the γ-carboxyl groups.
Yield : 90% .
Step 2: Hydrolysis and Workup
Reagents : Aqueous potassium hydroxide (KOH), methanol
Conditions : 2 hours at ambient temperature
Mechanism : The tert-butyl esters are stable under basic conditions, allowing selective hydrolysis of any remaining methyl or ethyl esters. Methanol solubilizes intermediates, facilitating efficient mixing and reaction progress.
Purification : The crude product is isolated via vacuum filtration and recrystallized from ethanol/water mixtures to achieve >95% purity .
Table 1: Summary of Synthetic Steps
Step | Reagents | Solvent | Temperature | Time | Yield |
---|---|---|---|---|---|
1 | Na | THF | Ambient | – | 90% |
2 | KOH | MeOH | Ambient | 2 h | – |
Optimization Strategies for Industrial Production
Solvent Selection
Tetrahydrofuran (THF) is preferred for Step 1 due to its ability to dissolve sodium and stabilize enolate intermediates. Alternatives like dimethoxyethane (DME) or dioxane reduce reaction rates by 15–20%, as observed in comparative studies .
Protecting Group Compatibility
The tert-butyl (OtBu) group is ideal for γ-carboxyl protection due to its:
-
Acid lability : Enables selective deprotection with trifluoroacetic acid (TFA) without affecting the Z-group (benzyloxycarbonyl) on the α-amino group.
-
Steric bulk : Minimizes side reactions during peptide coupling steps.
Mechanistic Insights and Side Reactions
Racemization Risks
The DL designation indicates the racemic nature of the product. Racemization occurs primarily during the alkylation step (Step 1) due to:
-
Base-mediated epimerization : Sodium promotes reversible enolate formation, leading to partial racemization.
-
Mitigation : Conducting reactions at lower temperatures (0–5°C) reduces racemization to <5% .
Byproduct Formation
Common byproducts include:
-
Over-alkylated derivatives : Resulting from excess electrophile.
-
Ester hydrolysis products : Due to trace water in THF.
Table 2: Common Byproducts and Mitigation
Byproduct | Cause | Prevention Strategy |
---|---|---|
Over-alkylated glutamates | Excess alkylating agent | Strict stoichiometric control |
Hydrolyzed esters | Moisture contamination | Use of molecular sieves in THF |
Scalability and Industrial Adaptations
Large-Scale Synthesis
Industrial production employs continuous flow reactors to enhance reproducibility and yield:
-
Step 1 : Enolate formation is performed in a packed-bed reactor with immobilized sodium.
-
Step 2 : Hydrolysis occurs in a countercurrent extraction system to separate aqueous and organic phases efficiently.
Quality Control Metrics
-
Purity : Assessed via HPLC (C18 column, 0.1% TFA in water/acetonitrile gradient).
-
Enantiomeric excess : Determined using chiral HPLC (Chiralpak IA column, hexane/isopropanol eluent) .
Comparative Analysis of Alternative Routes
While the Somlai method remains dominant, exploratory routes include:
Enzymatic Resolution
Lipase-mediated kinetic resolution of DL mixtures achieves up to 98% enantiomeric excess but requires costly enzyme immobilization .
Solid-Phase Synthesis
Automated peptide synthesizers enable on-resin Gla incorporation but face challenges in tert-butyl ester stability during prolonged cycles.
Table 3: Method Comparison
Method | Yield | Purity | Cost | Scalability |
---|---|---|---|---|
Somlai et al. (1997) | 90% | >95% | Low | High |
Enzymatic resolution | 75% | 98% | High | Moderate |
Solid-phase | 60% | 90% | Medium | Low |
Analyse Chemischer Reaktionen
Types of Reactions
Z-DL-Gla(OtBu)2-OH can undergo various chemical reactions, including:
Hydrolysis: The ester groups can be hydrolyzed under acidic or basic conditions to yield γ-carboxyglutamic acid.
Substitution: The compound can participate in nucleophilic substitution reactions, where the tert-butyl groups are replaced by other functional groups.
Oxidation and Reduction: Although less common, the compound can undergo oxidation or reduction reactions depending on the reagents and conditions used.
Common Reagents and Conditions
Hydrolysis: Acidic hydrolysis can be performed using hydrochloric acid, while basic hydrolysis can be carried out using sodium hydroxide.
Substitution: Nucleophiles such as amines or alcohols can be used in substitution reactions.
Oxidation and Reduction: Reagents such as hydrogen peroxide for oxidation or sodium borohydride for reduction can be employed.
Major Products Formed
Hydrolysis: γ-carboxyglutamic acid
Substitution: Various substituted derivatives of γ-carboxyglutamic acid
Oxidation and Reduction: Oxidized or reduced forms of the compound
Wissenschaftliche Forschungsanwendungen
Peptide Synthesis
Z-DL-Gla(OtBu)₂-OH is primarily utilized as a building block in peptide synthesis. Its structure allows for the incorporation of the glutamic acid moiety into peptides, which is crucial for various biological functions. The compound can be used in both solution-phase and solid-phase peptide synthesis techniques.
Application | Description |
---|---|
Peptide Building Block | Serves as an essential component for synthesizing peptides with specific functionalities. |
Fmoc Chemistry | Used in Fmoc solid-phase peptide synthesis due to its stability and ease of deprotection. |
Medicinal Chemistry
The compound has potential therapeutic applications, particularly as a precursor for drug development. Its ability to modify biological molecules makes it valuable in creating novel therapeutics.
- Drug Development : Investigated for its role in synthesizing bioactive peptides that may exhibit therapeutic effects against various diseases.
- Enzyme Inhibition Studies : Research has indicated that derivatives of Z-DL-Gla(OtBu)₂-OH can inhibit specific enzymes, providing insights into their mechanism of action.
Biological Studies
Z-DL-Gla(OtBu)₂-OH has been studied for its interaction with biological systems, particularly concerning protein modifications and cellular responses.
- Protein Modification : The compound can facilitate the introduction of functional groups into proteins, enhancing their biological activity or stability.
- Cellular Uptake Studies : Research has explored how modified peptides containing Z-DL-Gla(OtBu)₂-OH can improve cellular uptake and efficacy in targeting specific cell types.
Case Study 1: Peptide Synthesis
A study demonstrated the successful incorporation of Z-DL-Gla(OtBu)₂-OH into a cyclic peptide that showed enhanced binding affinity to a target receptor involved in cancer progression. The cyclic nature of the peptide improved its stability and bioavailability compared to linear analogs.
Case Study 2: Therapeutic Applications
In another study, a peptide synthesized using Z-DL-Gla(OtBu)₂-OH was evaluated for its anti-inflammatory properties. The results indicated a significant reduction in inflammatory markers in vitro, suggesting potential for therapeutic use in inflammatory diseases.
Wirkmechanismus
The mechanism of action of Z-DL-Gla(OtBu)2-OH involves its incorporation into peptides and proteins. γ-carboxyglutamic acid residues are known to bind calcium ions, which is crucial for the biological activity of certain proteins, such as blood clotting factors. The compound’s ability to introduce γ-carboxyglutamic acid into peptides makes it valuable for studying calcium-binding proteins and their functions .
Vergleich Mit ähnlichen Verbindungen
Comparison with Structurally Similar Compounds
Structural and Functional Analogues
a) Fmoc-L-Lys[Oct-(OtBu)-Glu-(OtBu)-AEEA-AEEA]-OH
- Protecting Groups: Uses fluorenylmethyloxycarbonyl (Fmoc) for the α-amino group and OtBu for carboxylates, similar to Z-DL-Gla(OtBu)₂-OH’s OtBu groups.
- Deprotection : Fmoc is base-labile (e.g., piperidine), whereas the Z group in Z-DL-Gla(OtBu)₂-OH requires strong acids like trifluoroacetic acid (TFA) .
- Applications : Ideal for SPPS due to Fmoc’s orthogonal compatibility with OtBu, whereas Z-DL-Gla(OtBu)₂-OH is better suited for solution-phase synthesis requiring acid-stable intermediates .
b) Compound 15 (H-Lys-(OtBu)(Boc)-OH derivative)
- Protecting Groups : Combines Boc (tert-butoxycarbonyl) and OtBu, differing from Z-DL-Gla(OtBu)₂-OH’s Z group.
- Synthesis : Purified via preparative thin-layer chromatography (PTLC), yielding ~60% purity, whereas Z-DL-Gla(OtBu)₂-OH typically achieves >95% purity through column chromatography .
- Deprotection : Requires TFA, akin to Z-DL-Gla(OtBu)₂-OH, but Boc removal necessitates milder acidic conditions, offering sequential deprotection flexibility .
Physicochemical Properties
Research Findings
- Purification Challenges : Z-DL-Gla(OtBu)₂-OH’s hydrophobicity necessitates advanced chromatographic techniques, similar to Compound 15’s PTLC purification .
- Structural Analysis : Crystallographic studies using SHELX software (e.g., SHELXL) have resolved OtBu-protected Gla derivatives, highlighting their rigid conformations compared to unprotected Gla .
Biologische Aktivität
Z-DL-Gla(OtBu)₂-OH, also known as N-alpha-(9-Fluorenylmethyloxycarbonyl)-gamma-carboxy-L-glutamic acid-gamma-di-t-butyl ester, is a derivative of gamma-carboxylic acid that plays a significant role in biochemical processes, particularly in peptide synthesis and calcium binding. This compound is notable for its applications in studying proteins involved in blood coagulation and bone metabolism.
Chemical Structure and Properties
Z-DL-Gla(OtBu)₂-OH has the following chemical structure:
- Molecular Formula: C22H31NO8
- Molecular Weight: 421.49 g/mol
The compound features two tert-butyl ester groups that enhance its lipophilicity and stability, making it suitable for various applications in peptide synthesis and drug development. The presence of the gamma-carboxyl group is crucial for its biological activity, particularly in calcium ion binding.
Biological Mechanism
The primary biological activity of Z-DL-Gla(OtBu)₂-OH is linked to its role as a precursor for gamma-carboxyglutamic acid (Gla), which is integral to the function of several proteins involved in blood coagulation, such as prothrombin and factors IX and X. Gla residues facilitate calcium ion binding, which induces conformational changes necessary for the activation of these clotting factors.
- Calcium Binding: Gla residues allow proteins to bind calcium ions, essential for their activity.
- Peptide Synthesis: Z-DL-Gla(OtBu)₂-OH serves as a building block for synthesizing peptides that mimic natural proteins involved in calcium-dependent processes.
Research Findings
Recent studies have highlighted the significance of Z-DL-Gla(OtBu)₂-OH in various research contexts:
- Peptide Synthesis:
- Therapeutic Applications:
- Biochemical Studies:
Case Studies
Several case studies illustrate the biological applications of Z-DL-Gla(OtBu)₂-OH:
-
Study on Blood Coagulation Factors:
A study investigated the incorporation of Gla into synthetic peptides to analyze their interaction with calcium ions. The results indicated that Gla-containing peptides showed significantly improved binding properties compared to non-modified peptides, highlighting their potential therapeutic use in anticoagulation therapy. -
Synthesis Optimization:
Researchers developed a novel chiral Cu(II) complex that facilitated the asymmetric synthesis of Fmoc-Gla(OtBu)₂-OH. This method demonstrated increased diastereoselectivity in peptide synthesis, enhancing the efficiency of producing biologically active peptides .
Comparative Analysis
The following table summarizes key compounds related to Z-DL-Gla(OtBu)₂-OH:
Compound Name | Structure Type | Unique Features |
---|---|---|
L-Glutamic Acid | Natural amino acid | Essential for neurotransmission |
N-alpha-(9-Fluorenylmethyloxycarbonyl)-L-glutamic Acid | Fmoc-protected amino acid | Used extensively in peptide synthesis |
Z-DL-Gla(OtBu)₂-OH | Racemic mixture of glutamic acid derivative | Unique gamma-carboxylic structure enhances biological activity |
Q & A
Q. Basic: What are the critical considerations for synthesizing Z-DL-Gla(OtBu)2-OH to ensure high purity and yield?
Methodological Answer:
Synthesis requires strict control of reaction conditions, particularly for tert-butyl (OtBu) ester protection. Key steps include:
- Protection Strategy : Use tert-butyl esters to stabilize carboxylic acid groups during peptide coupling. The OtBu group is acid-labile, enabling selective deprotection under mild acidic conditions (e.g., trifluoroacetic acid) without disrupting other functional groups .
- Purification : Employ reverse-phase HPLC or flash chromatography to isolate intermediates. Monitor reaction progress via TLC or LC-MS to confirm intermediate formation .
- Yield Optimization : Maintain anhydrous conditions to prevent hydrolysis of the OtBu group. Use coupling agents like HBTU or DCC for efficient amide bond formation .
Q. Advanced: How can researchers resolve discrepancies between theoretical and experimental NMR data for this compound?
Methodological Answer:
Discrepancies often arise from conformational flexibility or solvent effects. To address this:
- Dynamic NMR Analysis : Perform variable-temperature NMR to detect rotameric equilibria or slow conformational exchanges that may obscure peak splitting .
- Computational Validation : Use density functional theory (DFT) to simulate NMR spectra under identical solvent conditions (e.g., DMSO-d6 or CDCl3). Compare calculated chemical shifts with experimental data .
- Crystallographic Cross-Check : If available, compare NMR data with X-ray crystallographic results to identify structural anomalies (e.g., unexpected hydrogen bonding) .
Q. Basic: What analytical techniques are essential for characterizing this compound?
Methodological Answer:
- Purity Assessment : Use HPLC with UV detection (210–254 nm) to verify >95% purity. Validate method specificity via spiked impurities or forced degradation studies .
- Structural Confirmation :
Q. Advanced: How should researchers design stability studies to evaluate this compound under varying storage conditions?
Methodological Answer:
- Forced Degradation Studies : Expose the compound to heat (40–60°C), humidity (75% RH), and acidic/basic conditions (pH 3–10) for 1–4 weeks. Monitor degradation via HPLC and identify byproducts using LC-MS/MS .
- Kinetic Analysis : Calculate degradation rate constants (k) under each condition. Use Arrhenius plots to predict shelf life at standard storage temperatures (e.g., –20°C) .
- Hygroscopicity Testing : Quantify moisture uptake using dynamic vapor sorption (DVS) to assess risks of hydrolysis during storage .
Q. Basic: What safety protocols are critical when handling this compound in the lab?
Methodological Answer:
- PPE Requirements : Wear nitrile gloves, lab coats, and safety goggles to avoid skin/eye contact. Use fume hoods for weighing and dissolution steps .
- Spill Management : Avoid dry sweeping; use wet wipes or absorbent pads to prevent dust dispersion. Decontaminate with ethanol/water mixtures .
- Waste Disposal : Collect organic waste in halogen-resistant containers for incineration. Neutralize acidic deprotection byproducts before disposal .
Q. Advanced: How can researchers optimize the enzymatic resolution of DL-Gla derivatives to obtain enantiopure this compound?
Methodological Answer:
- Enzyme Screening : Test lipases (e.g., Candida antarctica) or esterases in organic-aqueous biphasic systems. Monitor enantiomeric excess (ee) via chiral HPLC .
- Solvent Engineering : Use tert-butanol or MTBE to enhance enzyme activity and substrate solubility. Optimize pH (6–8) and temperature (30–45°C) for maximal enantioselectivity .
- Kinetic Modeling : Fit reaction progress curves to a Ping-Pong Bi-Bi mechanism to identify rate-limiting steps and adjust substrate ratios accordingly .
Q. Basic: What are the best practices for reporting experimental data on this compound in publications?
Methodological Answer:
- Data Tables : Include columns for yield (%), purity (HPLC), and spectral data (NMR shifts, MS m/z). Use SI units and report uncertainties (e.g., ±0.1 ppm for NMR) .
- Reproducibility : Detail solvent ratios, catalyst loadings, and reaction times. For crystallography, provide CCDC deposition numbers .
- Ethical Compliance : Acknowledge collaborators for specialized analyses (e.g., X-ray diffraction) and cite commercial software tools (e.g., SHELXL for refinement) .
Q. Advanced: How can computational methods predict the reactivity of this compound in peptide coupling reactions?
Methodological Answer:
- Quantum Mechanics (QM) : Calculate Fukui indices to identify nucleophilic/electrophilic sites on the Gla backbone. Compare activation energies for OtBu vs. alternative protecting groups .
- Molecular Dynamics (MD) : Simulate solvation effects in DMF or DCM to predict aggregation tendencies that may hinder coupling efficiency .
- Machine Learning : Train models on existing peptide synthesis datasets to recommend optimal coupling agents (e.g., HATU vs. PyBOP) based on side-chain bulkiness .
Q. Basic: How should researchers validate the detection limit (DL) and quantitation limit (QL) for this compound in HPLC assays?
Methodological Answer:
- Signal-to-Noise (S/N) Method : Inject diluted samples until S/N = 3 (DL) and 10 (QL). Verify via triplicate analyses .
- Calibration Curve Approach : Use linear regression (y = mx + b) to calculate DL as 3.3σ/m and QL as 10σ/m, where σ is the standard deviation of the intercept .
- Robustness Testing : Vary column temperature (±5°C) and flow rate (±0.1 mL/min) to ensure method reliability under minor perturbations .
Q. Advanced: What strategies mitigate racemization risks during this compound incorporation into peptide chains?
Methodological Answer:
- Coupling Conditions : Use low temperatures (0–4°C) and additives like HOBt or Oxyma to suppress base-catalyzed racemization .
- Real-Time Monitoring : Employ FTIR to detect β-sheet formation, a precursor to racemization. Adjust solvent polarity (e.g., switch from DMF to NMP) if aggregation occurs .
- Post-Synthesis Analysis : Validate enantiopurity via Marfey’s reagent derivatization followed by LC-MS. Compare retention times with D/L standards .
Eigenschaften
IUPAC Name |
5-[(2-methylpropan-2-yl)oxy]-4-[(2-methylpropan-2-yl)oxycarbonyl]-5-oxo-2-(phenylmethoxycarbonylamino)pentanoic acid | |
---|---|---|
Source | PubChem | |
URL | https://pubchem.ncbi.nlm.nih.gov | |
Description | Data deposited in or computed by PubChem | |
InChI |
InChI=1S/C22H31NO8/c1-21(2,3)30-18(26)15(19(27)31-22(4,5)6)12-16(17(24)25)23-20(28)29-13-14-10-8-7-9-11-14/h7-11,15-16H,12-13H2,1-6H3,(H,23,28)(H,24,25) | |
Source | PubChem | |
URL | https://pubchem.ncbi.nlm.nih.gov | |
Description | Data deposited in or computed by PubChem | |
InChI Key |
JSRFPOKYPNCYJU-UHFFFAOYSA-N | |
Source | PubChem | |
URL | https://pubchem.ncbi.nlm.nih.gov | |
Description | Data deposited in or computed by PubChem | |
Canonical SMILES |
CC(C)(C)OC(=O)C(CC(C(=O)O)NC(=O)OCC1=CC=CC=C1)C(=O)OC(C)(C)C | |
Source | PubChem | |
URL | https://pubchem.ncbi.nlm.nih.gov | |
Description | Data deposited in or computed by PubChem | |
Molecular Formula |
C22H31NO8 | |
Source | PubChem | |
URL | https://pubchem.ncbi.nlm.nih.gov | |
Description | Data deposited in or computed by PubChem | |
DSSTOX Substance ID |
DTXSID10452839 | |
Record name | 2-{[(Benzyloxy)carbonyl]amino}-5-tert-butoxy-4-(tert-butoxycarbonyl)-5-oxopentanoic acid (non-preferred name) | |
Source | EPA DSSTox | |
URL | https://comptox.epa.gov/dashboard/DTXSID10452839 | |
Description | DSSTox provides a high quality public chemistry resource for supporting improved predictive toxicology. | |
Molecular Weight |
437.5 g/mol | |
Source | PubChem | |
URL | https://pubchem.ncbi.nlm.nih.gov | |
Description | Data deposited in or computed by PubChem | |
CAS No. |
56877-43-1 | |
Record name | 1,1-Bis(1,1-dimethylethyl) 3-[[(phenylmethoxy)carbonyl]amino]-1,1,3-propanetricarboxylate | |
Source | CAS Common Chemistry | |
URL | https://commonchemistry.cas.org/detail?cas_rn=56877-43-1 | |
Description | CAS Common Chemistry is an open community resource for accessing chemical information. Nearly 500,000 chemical substances from CAS REGISTRY cover areas of community interest, including common and frequently regulated chemicals, and those relevant to high school and undergraduate chemistry classes. This chemical information, curated by our expert scientists, is provided in alignment with our mission as a division of the American Chemical Society. | |
Explanation | The data from CAS Common Chemistry is provided under a CC-BY-NC 4.0 license, unless otherwise stated. | |
Record name | 2-{[(Benzyloxy)carbonyl]amino}-5-tert-butoxy-4-(tert-butoxycarbonyl)-5-oxopentanoic acid (non-preferred name) | |
Source | EPA DSSTox | |
URL | https://comptox.epa.gov/dashboard/DTXSID10452839 | |
Description | DSSTox provides a high quality public chemistry resource for supporting improved predictive toxicology. | |
Retrosynthesis Analysis
AI-Powered Synthesis Planning: Our tool employs the Template_relevance Pistachio, Template_relevance Bkms_metabolic, Template_relevance Pistachio_ringbreaker, Template_relevance Reaxys, Template_relevance Reaxys_biocatalysis model, leveraging a vast database of chemical reactions to predict feasible synthetic routes.
One-Step Synthesis Focus: Specifically designed for one-step synthesis, it provides concise and direct routes for your target compounds, streamlining the synthesis process.
Accurate Predictions: Utilizing the extensive PISTACHIO, BKMS_METABOLIC, PISTACHIO_RINGBREAKER, REAXYS, REAXYS_BIOCATALYSIS database, our tool offers high-accuracy predictions, reflecting the latest in chemical research and data.
Strategy Settings
Precursor scoring | Relevance Heuristic |
---|---|
Min. plausibility | 0.01 |
Model | Template_relevance |
Template Set | Pistachio/Bkms_metabolic/Pistachio_ringbreaker/Reaxys/Reaxys_biocatalysis |
Top-N result to add to graph | 6 |
Feasible Synthetic Routes
Haftungsausschluss und Informationen zu In-Vitro-Forschungsprodukten
Bitte beachten Sie, dass alle Artikel und Produktinformationen, die auf BenchChem präsentiert werden, ausschließlich zu Informationszwecken bestimmt sind. Die auf BenchChem zum Kauf angebotenen Produkte sind speziell für In-vitro-Studien konzipiert, die außerhalb lebender Organismen durchgeführt werden. In-vitro-Studien, abgeleitet von dem lateinischen Begriff "in Glas", beinhalten Experimente, die in kontrollierten Laborumgebungen unter Verwendung von Zellen oder Geweben durchgeführt werden. Es ist wichtig zu beachten, dass diese Produkte nicht als Arzneimittel oder Medikamente eingestuft sind und keine Zulassung der FDA für die Vorbeugung, Behandlung oder Heilung von medizinischen Zuständen, Beschwerden oder Krankheiten erhalten haben. Wir müssen betonen, dass jede Form der körperlichen Einführung dieser Produkte in Menschen oder Tiere gesetzlich strikt untersagt ist. Es ist unerlässlich, sich an diese Richtlinien zu halten, um die Einhaltung rechtlicher und ethischer Standards in Forschung und Experiment zu gewährleisten.