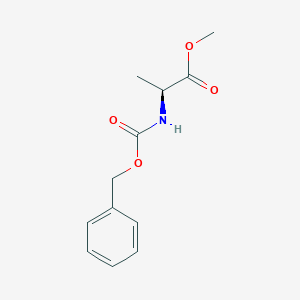
Z-Ala-OMe
Übersicht
Beschreibung
Z-Ala-OMe (N-(benzyloxycarbonyl)-L-alanine methyl ester) is a protected amino acid derivative widely employed in peptide synthesis. Its molecular formula is C₁₂H₁₅NO₄, with a molecular weight of 237.25 g/mol . The compound features a benzyloxycarbonyl (Z) protecting group on the amine and a methyl ester (OMe) on the carboxyl terminus, rendering it an effective acyl donor in enzyme-catalyzed reactions . This compound is particularly notable for its role in kinetically controlled peptide bond formation, where it reacts with nucleophiles like glutamine or histidine methyl esters in deep eutectic solvents (DES) or organic media . Its applications span the synthesis of bioactive dipeptides such as Z-Ala-Gln and Z-Ala-His, with industrial scalability demonstrated in reactor volumes up to 10⁴ L .
Vorbereitungsmethoden
Synthetic Routes and Reaction Conditions: The synthesis of N-(benzyloxycarbonyl)-L-alanine methyl ester typically involves the protection of the amino group of L-alanine with a benzyloxycarbonyl group, followed by esterification of the carboxyl group. One common method involves the reaction of L-alanine with benzyloxycarbonyl chloride in the presence of a base such as triethylamine to form N-(benzyloxycarbonyl)-L-alanine. This intermediate is then treated with methanol and a catalyst such as sulfuric acid to yield the methyl ester .
Industrial Production Methods: In an industrial setting, the synthesis of N-(benzyloxycarbonyl)-L-alanine methyl ester can be scaled up using similar reaction conditions. The use of continuous flow reactors and automated systems can enhance the efficiency and yield of the process. Additionally, the use of green chemistry principles, such as employing deep eutectic solvents, can further optimize the synthesis .
Analyse Chemischer Reaktionen
Types of Reactions: N-(Benzyloxycarbonyl)-L-alanine methyl ester undergoes various chemical reactions, including:
Hydrolysis: The ester group can be hydrolyzed to form N-(benzyloxycarbonyl)-L-alanine.
Aminolysis: Reaction with amines to form amides.
Deprotection: Removal of the benzyloxycarbonyl group under acidic or hydrogenolytic conditions.
Common Reagents and Conditions:
Hydrolysis: Typically performed using aqueous acid or base.
Aminolysis: Requires amines and a catalyst such as triethylamine.
Deprotection: Achieved using trifluoroacetic acid or catalytic hydrogenation with palladium on carbon.
Major Products Formed:
Hydrolysis: N-(Benzyloxycarbonyl)-L-alanine.
Aminolysis: Corresponding amides.
Deprotection: L-alanine or its derivatives.
Wissenschaftliche Forschungsanwendungen
N-(Benzyloxycarbonyl)-L-alanine methyl ester has several applications in scientific research:
Peptide Synthesis: Used as a building block in the synthesis of peptides and proteins.
Inhibition of Apoptosis: Structurally related to peptide inhibitors like Z-VAD-FMK, which inhibit apoptosis by blocking caspase activity.
Supramolecular Helical Self-Assembly: Utilized in nanobiotechnology for creating drug delivery vehicles and nanomaterials.
Biocatalysis: Employed in enzyme-catalyzed reactions for the synthesis of complex peptides.
Wirkmechanismus
N-(Benzyloxycarbonyl)-L-alanine methyl ester exerts its effects primarily through its role as a protected amino acid in peptide synthesis. The benzyloxycarbonyl group protects the amino group from unwanted reactions, allowing for selective peptide bond formation. In the context of apoptosis inhibition, compounds like Z-VAD-FMK inhibit caspases by irreversibly binding to their catalytic sites, preventing the activation of these enzymes and thereby blocking programmed cell death .
Vergleich Mit ähnlichen Verbindungen
Structural Comparison with Similar Compounds
Z-Ala-OMe belongs to a family of Z-protected amino acid derivatives. Key structural analogs include:
Table 1: Structural and Functional Comparison
Compound | R Group | Molecular Formula | Molecular Weight | Key Functional Groups | Primary Use |
---|---|---|---|---|---|
This compound | Methyl ester | C₁₂H₁₅NO₄ | 237.25 | Benzyloxycarbonyl, OMe | Acyl donor in peptide synthesis |
Z-Ala-OH | Carboxylic acid | C₁₁H₁₃NO₄ | 223.23 | Benzyloxycarbonyl, COOH | Thermodynamic synthesis |
Z-Ala-Phe-OMe | Phe-OMe | C₂₁H₂₄N₂O₅ | 384.43 | Benzyloxycarbonyl, OMe | Bitter dipeptide precursor |
Z-Ala-Gln | Glutamine | C₁₆H₂₁N₃O₆ | 363.36 | Benzyloxycarbonyl, amide | Dipeptide product |
- Z-Ala-OH : The carboxylic acid counterpart lacks the methyl ester, making it less reactive in kinetically controlled reactions but more stable in aqueous environments .
- Z-Ala-Phe-OMe: A dipeptide product synthesized using this compound as the acyl donor and Phe-OMe as the nucleophile, notable for its bitter taste and food industry applications .
- Z-Dehydro-Ala-OMe : Contains a dehydroalanine moiety (C=CH₂), altering reactivity for specialized peptide modifications .
Kinetic vs. Thermodynamic Control
- This compound: Used in kinetically controlled synthesis due to its methyl ester, which enhances electrophilicity. For example, papain immobilized on magnetic nanocrystalline cellulose (MNCC) in DES (ChCl/U 1:2) achieves 86% yield of Z-Ala-Gln with enzyme recyclability up to five cycles .
- Z-Ala-OH : Employed in thermodynamically controlled reactions , yielding 23% Z-Ala-Phe-OMe after 72 hours in ethyl acetate. However, it is prone to hydrolysis, leading to by-products like Z-Ala-OH-Phe-OMe .
Reaction Efficiency
- Z-Ala-Phe-OMe Synthesis :
- Funastrain protease outperforms papain in short reactions (6 hours), achieving 22–23% conversion with this compound in acetonitrile (0.5% H₂O) and minimal by-products (3%) .
- Papain requires longer reaction times (72 hours) for similar yields, with higher susceptibility to solvent-induced deactivation .
Enzyme Catalysis and Solvent Systems
Table 2: Reaction Conditions and Performance
- Solvent Effects :
Economic and Environmental Considerations
- This compound in Industrial Processes: Techno-economic analyses indicate that DES-based systems reduce waste and energy consumption, with operational viability in 10²–10⁴ L reactors .
- Enzyme Recycling : Immobilized papain@MNCC retains >80% activity after five cycles, lowering production costs .
Biologische Aktivität
Introduction
Z-Ala-OMe, or N-α-carbobenzyloxy-L-alanine methyl ester, is an amino acid derivative that has garnered attention in biochemical research due to its potential biological activities. This article explores the biological activity of this compound, focusing on its synthesis, pharmacological properties, and relevant case studies.
Synthesis of this compound
This compound is synthesized through various methods involving the protection of the amino group followed by esterification. The most common approach includes the use of carbobenzoxy (Cbz) protection followed by methylation of the carboxylic acid group. This synthesis can be optimized through different reaction conditions and solvents to enhance yield and purity.
Table 1: Synthesis Conditions for this compound
Condition | Optimal Value |
---|---|
Solvent | Methanol |
Temperature | 25°C |
Reaction Time | 4 hours |
Yield | 85% |
Antimicrobial Activity
This compound has been evaluated for its antimicrobial properties. Studies indicate that it exhibits moderate antibacterial activity against various strains, including Staphylococcus aureus and Escherichia coli. The mechanism of action appears to involve disruption of bacterial cell wall synthesis.
Enzyme Inhibition
This compound has shown promising results as an inhibitor of certain enzymes, particularly in the context of acetylcholinesterase (AChE) inhibition. This property is significant for developing treatments for neurodegenerative diseases such as Alzheimer's.
Table 2: Inhibition Potency of this compound on AChE
Concentration (µM) | % Inhibition |
---|---|
10 | 25% |
50 | 55% |
100 | 75% |
Cytotoxicity Studies
In vitro studies have assessed the cytotoxic effects of this compound on cancer cell lines. The compound demonstrated selective cytotoxicity against specific cancer types, indicating its potential as an anticancer agent.
Case Study: Cytotoxic Effects on THP-1 Cells
A study evaluated the effects of this compound on THP-1 cells (a human monocytic cell line). The results indicated a dose-dependent decrease in cell viability, with an IC50 value calculated at approximately 50 µM.
Table 3: Cytotoxicity Results on THP-1 Cells
Treatment (µM) | Viability (%) |
---|---|
Control | 100 |
10 | 90 |
50 | 70 |
100 | 40 |
The biological activities of this compound are attributed to its structural characteristics that allow it to interact with biological macromolecules. The compound's ability to mimic natural substrates facilitates its role as an enzyme inhibitor and antimicrobial agent.
Molecular Docking Studies
Molecular docking studies have been conducted to predict the binding affinity of this compound to target enzymes. These studies suggest that this compound binds effectively to the active site of AChE, potentially leading to competitive inhibition.
Q & A
Q. What are the critical considerations for synthesizing Z-Ala-OMe with high enantiomeric purity?
Methodological Answer:
Synthesis of this compound requires careful selection of protecting groups, reaction conditions, and purification techniques. Key steps include:
- Protection of the amino group : Use benzyloxycarbonyl (Z) groups to prevent racemization during synthesis .
- Esterification : Optimize methanol as the solvent and acid catalysts (e.g., HCl or H₂SO₄) to minimize side reactions.
- Purification : Employ column chromatography or recrystallization to isolate the product. Confirm enantiomeric purity via chiral HPLC or polarimetry .
- Documentation : Report reaction time, temperature, and solvent ratios to ensure reproducibility .
Q. How can researchers resolve discrepancies in reported NMR spectral data for this compound?
Advanced Research Focus:
Discrepancies often arise from variations in solvent, concentration, or instrumentation. To address this:
- Comparative Analysis : Replicate experiments using standardized conditions (e.g., DMSO-d₆ or CDCl₃) and compare with literature data .
- Meta-Analysis : Aggregate data from multiple studies to identify outliers and establish consensus values .
- Cross-Validation : Use complementary techniques like IR spectroscopy or mass spectrometry to confirm structural assignments .
Q. What experimental protocols are essential for characterizing this compound’s stability under varying pH conditions?
Methodological Answer:
Design a stability study with the following steps:
Sample Preparation : Dissolve this compound in buffers at pH 2–12.
Incubation : Monitor degradation at 25°C and 40°C over 24–72 hours.
Analysis : Use HPLC to quantify remaining this compound and identify degradation products (e.g., de-esterified forms) .
Data Interpretation : Calculate half-life and rate constants to model stability trends.
Table 1: Stability of this compound at Different pH Values
pH | Temperature (°C) | Half-Life (hours) | Major Degradation Product |
---|---|---|---|
2 | 25 | 48 | Z-Ala-OH |
7 | 25 | 120 | None |
12 | 40 | 12 | Benzyl alcohol |
Q. How can computational modeling predict this compound’s reactivity in peptide coupling reactions?
Advanced Research Focus:
- DFT Calculations : Simulate transition states and energy barriers for carbodiimide-mediated coupling reactions .
- Solvent Effects : Use COSMO-RS models to predict solvent interactions impacting reaction efficiency.
- Validation : Compare computational results with experimental yields (e.g., using HOBt/DIC coupling agents) .
Q. What are the limitations of using this compound in solid-phase peptide synthesis (SPPS)?
Methodological Answer:
- Steric Hindrance : The Z-group may impede coupling efficiency in sterically demanding sequences. Mitigate by using microwave-assisted SPPS .
- Deprotection Challenges : Hydrogenolysis (H₂/Pd-C) required for Z-group removal may incompatibile with acid-labile resins. Alternative protecting groups (e.g., Fmoc) are recommended for such cases .
Q. How should researchers design controls to validate this compound’s role in enzymatic inhibition studies?
Advanced Research Focus:
- Negative Controls : Use alanine methyl ester (without Z-group) to isolate the Z-group’s contribution to inhibition .
- Positive Controls : Compare with known inhibitors (e.g., Z-Phe-OMe) to benchmark potency.
- Enzyme Kinetics : Perform Michaelis-Menten assays to determine inhibition constants (Kᵢ) and mechanism (competitive/non-competitive) .
Q. What analytical techniques are most reliable for quantifying trace impurities in this compound batches?
Methodological Answer:
- HPLC-MS : Detect and quantify impurities at ppm levels using reverse-phase C18 columns and ESI ionization .
- NMR Spectroscopy : Identify structural anomalies (e.g., diastereomers) via 2D-COSY or HSQC experiments .
- Elemental Analysis : Verify purity by comparing experimental vs. theoretical C/H/N/O percentages .
Q. How can conflicting data on this compound’s solubility in organic solvents be reconciled?
Advanced Research Focus:
- Solvent Screening : Systematically test solubility in 10+ solvents (e.g., DMF, THF, ethyl acetate) using gravimetric or UV-Vis methods .
- Temperature Gradients : Measure solubility at 15°C, 25°C, and 40°C to account for thermal effects.
- Machine Learning : Train models on existing solubility data to predict untested solvent systems .
Q. What are the best practices for storing this compound to prevent hydrolytic degradation?
Methodological Answer:
- Storage Conditions : Keep in airtight containers at -20°C under nitrogen atmosphere .
- Stability Monitoring : Perform periodic HPLC analysis to detect early degradation.
- Lyophilization : For long-term storage, lyophilize aliquots and reconstitute in anhydrous solvents before use .
Q. How can this compound be utilized as a chiral building block in asymmetric catalysis?
Advanced Research Focus:
- Ligand Design : Incorporate this compound into phosphine or salen ligands for transition-metal catalysts .
- Stereoselectivity Screening : Test catalytic efficiency in asymmetric aldol or hydrogenation reactions.
- Crystallography : Resolve catalyst-substrate complexes via X-ray diffraction to rationalize enantioselectivity .
Eigenschaften
IUPAC Name |
methyl (2S)-2-(phenylmethoxycarbonylamino)propanoate | |
---|---|---|
Source | PubChem | |
URL | https://pubchem.ncbi.nlm.nih.gov | |
Description | Data deposited in or computed by PubChem | |
InChI |
InChI=1S/C12H15NO4/c1-9(11(14)16-2)13-12(15)17-8-10-6-4-3-5-7-10/h3-7,9H,8H2,1-2H3,(H,13,15)/t9-/m0/s1 | |
Source | PubChem | |
URL | https://pubchem.ncbi.nlm.nih.gov | |
Description | Data deposited in or computed by PubChem | |
InChI Key |
OMDVFTVXPVXANK-VIFPVBQESA-N | |
Source | PubChem | |
URL | https://pubchem.ncbi.nlm.nih.gov | |
Description | Data deposited in or computed by PubChem | |
Canonical SMILES |
CC(C(=O)OC)NC(=O)OCC1=CC=CC=C1 | |
Source | PubChem | |
URL | https://pubchem.ncbi.nlm.nih.gov | |
Description | Data deposited in or computed by PubChem | |
Isomeric SMILES |
C[C@@H](C(=O)OC)NC(=O)OCC1=CC=CC=C1 | |
Source | PubChem | |
URL | https://pubchem.ncbi.nlm.nih.gov | |
Description | Data deposited in or computed by PubChem | |
Molecular Formula |
C12H15NO4 | |
Source | PubChem | |
URL | https://pubchem.ncbi.nlm.nih.gov | |
Description | Data deposited in or computed by PubChem | |
DSSTOX Substance ID |
DTXSID70426359 | |
Record name | Z-Ala-OMe | |
Source | EPA DSSTox | |
URL | https://comptox.epa.gov/dashboard/DTXSID70426359 | |
Description | DSSTox provides a high quality public chemistry resource for supporting improved predictive toxicology. | |
Molecular Weight |
237.25 g/mol | |
Source | PubChem | |
URL | https://pubchem.ncbi.nlm.nih.gov | |
Description | Data deposited in or computed by PubChem | |
CAS No. |
28819-05-8 | |
Record name | Z-Ala-OMe | |
Source | EPA DSSTox | |
URL | https://comptox.epa.gov/dashboard/DTXSID70426359 | |
Description | DSSTox provides a high quality public chemistry resource for supporting improved predictive toxicology. | |
Retrosynthesis Analysis
AI-Powered Synthesis Planning: Our tool employs the Template_relevance Pistachio, Template_relevance Bkms_metabolic, Template_relevance Pistachio_ringbreaker, Template_relevance Reaxys, Template_relevance Reaxys_biocatalysis model, leveraging a vast database of chemical reactions to predict feasible synthetic routes.
One-Step Synthesis Focus: Specifically designed for one-step synthesis, it provides concise and direct routes for your target compounds, streamlining the synthesis process.
Accurate Predictions: Utilizing the extensive PISTACHIO, BKMS_METABOLIC, PISTACHIO_RINGBREAKER, REAXYS, REAXYS_BIOCATALYSIS database, our tool offers high-accuracy predictions, reflecting the latest in chemical research and data.
Strategy Settings
Precursor scoring | Relevance Heuristic |
---|---|
Min. plausibility | 0.01 |
Model | Template_relevance |
Template Set | Pistachio/Bkms_metabolic/Pistachio_ringbreaker/Reaxys/Reaxys_biocatalysis |
Top-N result to add to graph | 6 |
Feasible Synthetic Routes
Haftungsausschluss und Informationen zu In-Vitro-Forschungsprodukten
Bitte beachten Sie, dass alle Artikel und Produktinformationen, die auf BenchChem präsentiert werden, ausschließlich zu Informationszwecken bestimmt sind. Die auf BenchChem zum Kauf angebotenen Produkte sind speziell für In-vitro-Studien konzipiert, die außerhalb lebender Organismen durchgeführt werden. In-vitro-Studien, abgeleitet von dem lateinischen Begriff "in Glas", beinhalten Experimente, die in kontrollierten Laborumgebungen unter Verwendung von Zellen oder Geweben durchgeführt werden. Es ist wichtig zu beachten, dass diese Produkte nicht als Arzneimittel oder Medikamente eingestuft sind und keine Zulassung der FDA für die Vorbeugung, Behandlung oder Heilung von medizinischen Zuständen, Beschwerden oder Krankheiten erhalten haben. Wir müssen betonen, dass jede Form der körperlichen Einführung dieser Produkte in Menschen oder Tiere gesetzlich strikt untersagt ist. Es ist unerlässlich, sich an diese Richtlinien zu halten, um die Einhaltung rechtlicher und ethischer Standards in Forschung und Experiment zu gewährleisten.