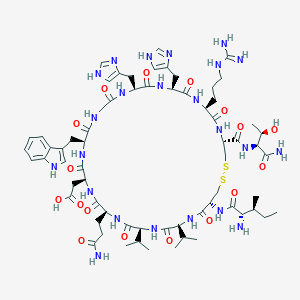
Compstatin
Übersicht
Beschreibung
Compstatin is a cyclic tridecapeptide that acts as a potent and selective inhibitor of the complement component C3. It was originally discovered through phage-display libraries and has shown significant potential in clinical applications due to its ability to modulate the complement system, which is a crucial part of the immune response .
Wissenschaftliche Forschungsanwendungen
Compstatin hat eine breite Palette von Anwendungen in der wissenschaftlichen Forschung, insbesondere in den Bereichen Immunologie und Medizin:
Immunologie: this compound wird zur Untersuchung des Komplementsystems verwendet, eines Teils des Immunsystems, der die Fähigkeit von Antikörpern und phagozytischen Zellen zur Beseitigung von Krankheitserregern verbessert.
Transplantation: This compound wurde in experimentellen Modellen verwendet, um komplementvermittelte Schäden bei Organtransplantationen zu verhindern.
Entzündung: This compound hat in verschiedenen Entzündungsmodellen entzündungshemmende Wirkungen gezeigt.
Wirkmechanismus
This compound übt seine Wirkungen aus, indem es an die Komplementkomponente C3 bindet und deren Aktivierung verhindert. Diese Hemmung blockiert die Spaltung von C3 in C3a und C3b, die für die nachgeschaltete Aktivierung der Komplementkaskade entscheidend sind. Durch die Hemmung der C3-Aktivierung moduliert this compound das Komplementsystem effektiv und verhindert die Bildung proinflammatorischer und zellschädigender Komponenten .
Vorbereitungsmethoden
Compstatin is synthesized using solid-phase peptide synthesis (SPPS), a method that allows for the sequential addition of amino acids to a growing peptide chain. The synthesis involves the following steps:
Solid-Phase Peptide Synthesis (SPPS): The peptide is assembled on a solid support, typically a resin, using protected amino acids. The amino acids are added one by one in a specific sequence.
Cyclization: After the linear peptide is synthesized, it undergoes cyclization to form the cyclic structure of this compound. This step is crucial for its biological activity.
Purification: The synthesized peptide is purified using high-performance liquid chromatography (HPLC) to remove any impurities.
Characterization: The final product is characterized using techniques such as mass spectrometry and nuclear magnetic resonance (NMR) spectroscopy to confirm its structure and purity.
Analyse Chemischer Reaktionen
Compstatin unterliegt während seiner Synthese hauptsächlich der Peptidbindungsbildung und Cyclisierung. Es unterliegt normalerweise nicht Oxidations-, Reduktions- oder Substitutionsreaktionen als Teil seiner Standardherstellung. Das Hauptprodukt, das aus diesen Reaktionen entsteht, ist das zyklische Tridecapeptid this compound selbst .
Wirkmechanismus
Compstatin exerts its effects by binding to the complement component C3 and preventing its activation. This inhibition blocks the cleavage of C3 into C3a and C3b, which are crucial for the downstream activation of the complement cascade. By inhibiting C3 activation, this compound effectively modulates the complement system and prevents the formation of pro-inflammatory and cell-damaging components .
Vergleich Mit ähnlichen Verbindungen
Compstatin ist einzigartig in seiner Fähigkeit, C3, eine zentrale Komponente des Komplementsystems, selektiv zu hemmen. Zu ähnlichen Verbindungen gehören:
Ravulizumab: Ein weiterer C5-Inhibitor mit einer längeren Halbwertszeit im Vergleich zu Eculizumab.
Pegcetacoplan: Ein Derivat von this compound, das für die Behandlung der paroxysmalen nächtlichen Hämoglobinurie zugelassen ist.
Die Einzigartigkeit von this compound liegt in seiner Fähigkeit, C3 anzugreifen, wodurch eine umfassendere Kontrolle des Komplementsystems im Vergleich zu C5-Inhibitoren ermöglicht wird .
Eigenschaften
IUPAC Name |
2-[(4R,7S,10S,13S,19S,22S,25S,28S,31S,34R)-4-[[(2S,3R)-1-amino-3-hydroxy-1-oxobutan-2-yl]carbamoyl]-34-[[(2S,3S)-2-amino-3-methylpentanoyl]amino]-25-(3-amino-3-oxopropyl)-7-[3-(diaminomethylideneamino)propyl]-10,13-bis(1H-imidazol-5-ylmethyl)-19-(1H-indol-3-ylmethyl)-6,9,12,15,18,21,24,27,30,33-decaoxo-28,31-di(propan-2-yl)-1,2-dithia-5,8,11,14,17,20,23,26,29,32-decazacyclopentatriacont-22-yl]acetic acid | |
---|---|---|
Source | PubChem | |
URL | https://pubchem.ncbi.nlm.nih.gov | |
Description | Data deposited in or computed by PubChem | |
InChI |
InChI=1S/C66H99N23O17S2/c1-8-32(6)50(68)63(104)86-46-27-108-107-26-45(62(103)89-53(33(7)90)54(69)95)85-56(97)39(14-11-17-74-66(70)71)80-59(100)43(20-36-24-73-29-78-36)83-58(99)42(19-35-23-72-28-77-35)79-48(92)25-76-55(96)41(18-34-22-75-38-13-10-9-12-37(34)38)82-60(101)44(21-49(93)94)84-57(98)40(15-16-47(67)91)81-64(105)51(30(2)3)88-65(106)52(31(4)5)87-61(46)102/h9-10,12-13,22-24,28-33,39-46,50-53,75,90H,8,11,14-21,25-27,68H2,1-7H3,(H2,67,91)(H2,69,95)(H,72,77)(H,73,78)(H,76,96)(H,79,92)(H,80,100)(H,81,105)(H,82,101)(H,83,99)(H,84,98)(H,85,97)(H,86,104)(H,87,102)(H,88,106)(H,89,103)(H,93,94)(H4,70,71,74)/t32-,33+,39-,40-,41-,42-,43-,44-,45-,46-,50-,51-,52-,53-/m0/s1 | |
Source | PubChem | |
URL | https://pubchem.ncbi.nlm.nih.gov | |
Description | Data deposited in or computed by PubChem | |
InChI Key |
RDTRHBCZFDCUPW-KWICJJCGSA-N | |
Source | PubChem | |
URL | https://pubchem.ncbi.nlm.nih.gov | |
Description | Data deposited in or computed by PubChem | |
Canonical SMILES |
CCC(C)C(C(=O)NC1CSSCC(NC(=O)C(NC(=O)C(NC(=O)C(NC(=O)CNC(=O)C(NC(=O)C(NC(=O)C(NC(=O)C(NC(=O)C(NC1=O)C(C)C)C(C)C)CCC(=O)N)CC(=O)O)CC2=CNC3=CC=CC=C32)CC4=CN=CN4)CC5=CN=CN5)CCCN=C(N)N)C(=O)NC(C(C)O)C(=O)N)N | |
Source | PubChem | |
URL | https://pubchem.ncbi.nlm.nih.gov | |
Description | Data deposited in or computed by PubChem | |
Isomeric SMILES |
CC[C@H](C)[C@@H](C(=O)N[C@H]1CSSC[C@H](NC(=O)[C@@H](NC(=O)[C@@H](NC(=O)[C@@H](NC(=O)CNC(=O)[C@@H](NC(=O)[C@@H](NC(=O)[C@@H](NC(=O)[C@@H](NC(=O)[C@@H](NC1=O)C(C)C)C(C)C)CCC(=O)N)CC(=O)O)CC2=CNC3=CC=CC=C32)CC4=CN=CN4)CC5=CN=CN5)CCCN=C(N)N)C(=O)N[C@@H]([C@@H](C)O)C(=O)N)N | |
Source | PubChem | |
URL | https://pubchem.ncbi.nlm.nih.gov | |
Description | Data deposited in or computed by PubChem | |
Molecular Formula |
C66H99N23O17S2 | |
Source | PubChem | |
URL | https://pubchem.ncbi.nlm.nih.gov | |
Description | Data deposited in or computed by PubChem | |
Molecular Weight |
1550.8 g/mol | |
Source | PubChem | |
URL | https://pubchem.ncbi.nlm.nih.gov | |
Description | Data deposited in or computed by PubChem | |
Retrosynthesis Analysis
AI-Powered Synthesis Planning: Our tool employs the Template_relevance Pistachio, Template_relevance Bkms_metabolic, Template_relevance Pistachio_ringbreaker, Template_relevance Reaxys, Template_relevance Reaxys_biocatalysis model, leveraging a vast database of chemical reactions to predict feasible synthetic routes.
One-Step Synthesis Focus: Specifically designed for one-step synthesis, it provides concise and direct routes for your target compounds, streamlining the synthesis process.
Accurate Predictions: Utilizing the extensive PISTACHIO, BKMS_METABOLIC, PISTACHIO_RINGBREAKER, REAXYS, REAXYS_BIOCATALYSIS database, our tool offers high-accuracy predictions, reflecting the latest in chemical research and data.
Strategy Settings
Precursor scoring | Relevance Heuristic |
---|---|
Min. plausibility | 0.01 |
Model | Template_relevance |
Template Set | Pistachio/Bkms_metabolic/Pistachio_ringbreaker/Reaxys/Reaxys_biocatalysis |
Top-N result to add to graph | 6 |
Feasible Synthetic Routes
Haftungsausschluss und Informationen zu In-Vitro-Forschungsprodukten
Bitte beachten Sie, dass alle Artikel und Produktinformationen, die auf BenchChem präsentiert werden, ausschließlich zu Informationszwecken bestimmt sind. Die auf BenchChem zum Kauf angebotenen Produkte sind speziell für In-vitro-Studien konzipiert, die außerhalb lebender Organismen durchgeführt werden. In-vitro-Studien, abgeleitet von dem lateinischen Begriff "in Glas", beinhalten Experimente, die in kontrollierten Laborumgebungen unter Verwendung von Zellen oder Geweben durchgeführt werden. Es ist wichtig zu beachten, dass diese Produkte nicht als Arzneimittel oder Medikamente eingestuft sind und keine Zulassung der FDA für die Vorbeugung, Behandlung oder Heilung von medizinischen Zuständen, Beschwerden oder Krankheiten erhalten haben. Wir müssen betonen, dass jede Form der körperlichen Einführung dieser Produkte in Menschen oder Tiere gesetzlich strikt untersagt ist. Es ist unerlässlich, sich an diese Richtlinien zu halten, um die Einhaltung rechtlicher und ethischer Standards in Forschung und Experiment zu gewährleisten.