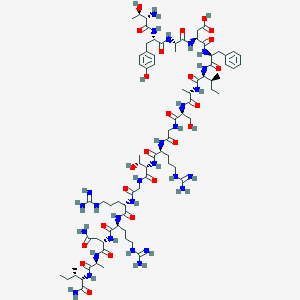
Tyadfiasgrtgrrnai-nh2
- Klicken Sie auf QUICK INQUIRY, um ein Angebot von unserem Expertenteam zu erhalten.
- Mit qualitativ hochwertigen Produkten zu einem WETTBEWERBSFÄHIGEN Preis können Sie sich mehr auf Ihre Forschung konzentrieren.
Übersicht
Beschreibung
Wissenschaftliche Forschungsanwendungen
Chemistry: Used as a tool to study protein-protein interactions and enzyme kinetics.
Biology: Helps in understanding signal transduction pathways involving PKA.
Medicine: Investigated for its potential therapeutic effects in conditions like morphine tolerance and long-term potentiation at hippocampal synapses.
Industry: Utilized in the development of biochemical assays and diagnostic tools .
Wirkmechanismus
Target of Action
Mode of Action
This compound is a synthetic peptide inhibitor of PKA . It is derived from the heat-stable PKA inhibitor protein PKI . The compound retains high potency for PKA inhibition . Both the arginine-containing pseudosubstrate site of the PKI peptide in its COOH terminus and the residue Phe 10 in NH2-terminal portion are required for this high affinity binding .
Biochemical Pathways
The compound inhibits the PKA, a key enzyme in the cAMP signaling pathway . This pathway plays a crucial role in multiple cellular processes, including cell proliferation, differentiation, and apoptosis .
Result of Action
The inhibition of PKA by this compound can significantly alter the cellular processes controlled by the cAMP signaling pathway . The exact molecular and cellular effects would depend on the specific cell type and context.
Vorbereitungsmethoden
Synthetic Routes and Reaction Conditions
Industrial Production Methods
Analyse Chemischer Reaktionen
Types of Reactions
Common Reagents and Conditions
Peptide Bond Formation: Activating agents like HBTU or DIC.
Hydrolysis: Acidic or basic conditions.
Oxidation: Oxidizing agents such as hydrogen peroxide.
Reduction: Reducing agents like dithiothreitol (DTT).
Major Products
Vergleich Mit ähnlichen Verbindungen
Similar Compounds
PKI (5-24) amide: Another synthetic peptide inhibitor of PKA.
H-89: A small molecule inhibitor of PKA.
KT5720: A selective inhibitor of PKA.
Uniqueness
Eigenschaften
IUPAC Name |
(3S)-4-[[(2S)-1-[[(2S,3S)-1-[[(2S)-1-[[(2S)-1-[[2-[[(2S)-1-[[(2S,3R)-1-[[2-[[(2S)-1-[[(2S)-1-[[(2S)-4-amino-1-[[(2S)-1-[[(2S,3S)-1-amino-3-methyl-1-oxopentan-2-yl]amino]-1-oxopropan-2-yl]amino]-1,4-dioxobutan-2-yl]amino]-5-carbamimidamido-1-oxopentan-2-yl]amino]-5-carbamimidamido-1-oxopentan-2-yl]amino]-2-oxoethyl]amino]-3-hydroxy-1-oxobutan-2-yl]amino]-5-carbamimidamido-1-oxopentan-2-yl]amino]-2-oxoethyl]amino]-3-hydroxy-1-oxopropan-2-yl]amino]-1-oxopropan-2-yl]amino]-3-methyl-1-oxopentan-2-yl]amino]-1-oxo-3-phenylpropan-2-yl]amino]-3-[[(2S)-2-[[(2S)-2-[[(2S,3R)-2-amino-3-hydroxybutanoyl]amino]-3-(4-hydroxyphenyl)propanoyl]amino]propanoyl]amino]-4-oxobutanoic acid |
Source
|
---|---|---|
Source | PubChem | |
URL | https://pubchem.ncbi.nlm.nih.gov | |
Description | Data deposited in or computed by PubChem | |
InChI |
InChI=1S/C80H130N28O24/c1-10-37(3)60(63(83)118)106-66(121)41(7)96-72(127)52(32-55(81)113)103-69(124)49(22-17-29-92-80(88)89)100-68(123)47(20-15-27-90-78(84)85)98-57(115)35-94-76(131)62(43(9)111)108-70(125)48(21-16-28-91-79(86)87)99-56(114)34-93-67(122)54(36-109)105-65(120)40(6)97-77(132)61(38(4)11-2)107-74(129)51(30-44-18-13-12-14-19-44)102-73(128)53(33-58(116)117)101-64(119)39(5)95-71(126)50(104-75(130)59(82)42(8)110)31-45-23-25-46(112)26-24-45/h12-14,18-19,23-26,37-43,47-54,59-62,109-112H,10-11,15-17,20-22,27-36,82H2,1-9H3,(H2,81,113)(H2,83,118)(H,93,122)(H,94,131)(H,95,126)(H,96,127)(H,97,132)(H,98,115)(H,99,114)(H,100,123)(H,101,119)(H,102,128)(H,103,124)(H,104,130)(H,105,120)(H,106,121)(H,107,129)(H,108,125)(H,116,117)(H4,84,85,90)(H4,86,87,91)(H4,88,89,92)/t37-,38-,39-,40-,41-,42+,43+,47-,48-,49-,50-,51-,52-,53-,54-,59-,60-,61-,62-/m0/s1 |
Source
|
Source | PubChem | |
URL | https://pubchem.ncbi.nlm.nih.gov | |
Description | Data deposited in or computed by PubChem | |
InChI Key |
VAKHFAFLRUNHLQ-PEBJKXEYSA-N |
Source
|
Source | PubChem | |
URL | https://pubchem.ncbi.nlm.nih.gov | |
Description | Data deposited in or computed by PubChem | |
Canonical SMILES |
CCC(C)C(C(=O)N)NC(=O)C(C)NC(=O)C(CC(=O)N)NC(=O)C(CCCNC(=N)N)NC(=O)C(CCCNC(=N)N)NC(=O)CNC(=O)C(C(C)O)NC(=O)C(CCCNC(=N)N)NC(=O)CNC(=O)C(CO)NC(=O)C(C)NC(=O)C(C(C)CC)NC(=O)C(CC1=CC=CC=C1)NC(=O)C(CC(=O)O)NC(=O)C(C)NC(=O)C(CC2=CC=C(C=C2)O)NC(=O)C(C(C)O)N |
Source
|
Source | PubChem | |
URL | https://pubchem.ncbi.nlm.nih.gov | |
Description | Data deposited in or computed by PubChem | |
Isomeric SMILES |
CC[C@H](C)[C@@H](C(=O)N)NC(=O)[C@H](C)NC(=O)[C@H](CC(=O)N)NC(=O)[C@H](CCCNC(=N)N)NC(=O)[C@H](CCCNC(=N)N)NC(=O)CNC(=O)[C@H]([C@@H](C)O)NC(=O)[C@H](CCCNC(=N)N)NC(=O)CNC(=O)[C@H](CO)NC(=O)[C@H](C)NC(=O)[C@H]([C@@H](C)CC)NC(=O)[C@H](CC1=CC=CC=C1)NC(=O)[C@H](CC(=O)O)NC(=O)[C@H](C)NC(=O)[C@H](CC2=CC=C(C=C2)O)NC(=O)[C@H]([C@@H](C)O)N |
Source
|
Source | PubChem | |
URL | https://pubchem.ncbi.nlm.nih.gov | |
Description | Data deposited in or computed by PubChem | |
Molecular Formula |
C80H130N28O24 |
Source
|
Source | PubChem | |
URL | https://pubchem.ncbi.nlm.nih.gov | |
Description | Data deposited in or computed by PubChem | |
Molecular Weight |
1868.1 g/mol |
Source
|
Source | PubChem | |
URL | https://pubchem.ncbi.nlm.nih.gov | |
Description | Data deposited in or computed by PubChem | |
Q1: How does PKA inhibitor fragment (6-22) amide interact with its target, and what are the downstream effects of this interaction?
A: PKA inhibitor fragment (6-22) amide acts as a competitive inhibitor of Protein Kinase A (PKA), specifically targeting the catalytic subunit of the enzyme. [, ] This interaction prevents the binding of ATP and subsequent phosphorylation of downstream substrates, effectively inhibiting PKA activity. [] Consequently, this inhibition can modulate a range of cellular processes, including ion channel activity, neurotransmitter release, and cellular responses to hypoxia. [, ]
Q2: What is the role of PKA inhibitor fragment (6-22) amide in studying adenosine signaling pathways?
A: Research has shown that PKA inhibitor fragment (6-22) amide can be used to dissect the role of PKA in adenosine signaling. For example, a study using rat PC12 cells demonstrated that the modulatory effects of adenosine on hypoxia-induced membrane responses were abolished by intracellular application of PKA inhibitor fragment (6-22) amide. [] This suggests that the PKA pathway is crucial for adenosine-mediated modulation of membrane excitability under hypoxic conditions. []
Q3: Are there any studies indicating the use of PKA inhibitor fragment (6-22) amide in understanding neurotransmitter release mechanisms?
A: Yes, PKA inhibitor fragment (6-22) amide has been employed to investigate the intricate mechanisms of neurotransmitter release. A study focusing on glycine release in rat spinal cord slices revealed that direct injection of PKA inhibitor fragment (6-22) amide into neurons significantly impacted the amplitude of evoked inhibitory postsynaptic currents (eIPSCs). [] This effect indicated that PKA activity plays a crucial role in regulating the presynaptic release of glycine. []
Haftungsausschluss und Informationen zu In-Vitro-Forschungsprodukten
Bitte beachten Sie, dass alle Artikel und Produktinformationen, die auf BenchChem präsentiert werden, ausschließlich zu Informationszwecken bestimmt sind. Die auf BenchChem zum Kauf angebotenen Produkte sind speziell für In-vitro-Studien konzipiert, die außerhalb lebender Organismen durchgeführt werden. In-vitro-Studien, abgeleitet von dem lateinischen Begriff "in Glas", beinhalten Experimente, die in kontrollierten Laborumgebungen unter Verwendung von Zellen oder Geweben durchgeführt werden. Es ist wichtig zu beachten, dass diese Produkte nicht als Arzneimittel oder Medikamente eingestuft sind und keine Zulassung der FDA für die Vorbeugung, Behandlung oder Heilung von medizinischen Zuständen, Beschwerden oder Krankheiten erhalten haben. Wir müssen betonen, dass jede Form der körperlichen Einführung dieser Produkte in Menschen oder Tiere gesetzlich strikt untersagt ist. Es ist unerlässlich, sich an diese Richtlinien zu halten, um die Einhaltung rechtlicher und ethischer Standards in Forschung und Experiment zu gewährleisten.