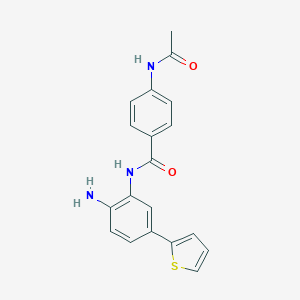
Merk60
Übersicht
Beschreibung
BRD6929 is a histone deacetylase 1 (HDAC1) and HDAC2 inhibitor (IC50s = 0.04 and 0.1 µM, respectively, for the recombinant human enzymes). It is selective for HDAC1 and HDAC2 over HDAC3 and HDAC8 (IC50s = >20 µM for both). BRD6929 inhibits proliferation of HCT116 human colon cancer cells but not non-cancerous human mammary epithelial cells (HMECs; IC50s = 4 and >50 µM, respectively).
Novel Selective Inhibitor of HDAC1 and HDAC2
4-acetamido-N-(2-amino-5-thiophen-2-ylphenyl)benzamide is a member of benzamides.
Wissenschaftliche Forschungsanwendungen
Mood-Related Behavioral Model Research
Due to its brain-penetrant properties, BRD-6929 has potential applications in mood-related behavioral model research. Its ability to alter gene expression in brain circuits makes it a valuable compound for studying neurological functions and disorders .
Latent HIV-1 Research
BRD-6929 has been shown to potentiate the efficacy of certain treatments against latent HIV-1. This is due to its selective inhibition of HDAC1 and HDAC2, which are implicated in the latency of HIV-1 .
Wirkmechanismus
Mode of Action
BRD-6929 interacts with its targets, HDAC1 and HDAC2, by binding to them with high affinity . The Ki values, which represent the binding affinity, are 0.2 nM for HDAC1 and 1.5 nM for HDAC2 . This interaction results in the inhibition of these enzymes, thereby affecting the acetylation status of histones and other proteins .
Biochemical Pathways
The inhibition of HDAC1 and HDAC2 by BRD-6929 affects the epigenetic regulation of gene expression . This can alter the transcription of various genes, including oncogenes and tumor suppressor genes . Moreover, BRD-6929 can induce a dose-dependent acetylation of H4K12ac in cultured neurons .
Pharmacokinetics
It is noted that brd-6929 is brain-penetrant , which suggests that it can cross the blood-brain barrier and exert its effects in the brain.
Result of Action
It causes an increase in H4K12 acetylation in brain region-specific primary cultures . Additionally, BRD-6929 induces a significant increase in H2B acetylation in primary neuronal cell cultures .
Action Environment
Given that brd-6929 is brain-penetrant , it can be inferred that the brain environment could potentially influence its action and efficacy.
Eigenschaften
IUPAC Name |
4-acetamido-N-(2-amino-5-thiophen-2-ylphenyl)benzamide | |
---|---|---|
Source | PubChem | |
URL | https://pubchem.ncbi.nlm.nih.gov | |
Description | Data deposited in or computed by PubChem | |
InChI |
InChI=1S/C19H17N3O2S/c1-12(23)21-15-7-4-13(5-8-15)19(24)22-17-11-14(6-9-16(17)20)18-3-2-10-25-18/h2-11H,20H2,1H3,(H,21,23)(H,22,24) | |
Source | PubChem | |
URL | https://pubchem.ncbi.nlm.nih.gov | |
Description | Data deposited in or computed by PubChem | |
InChI Key |
ABZSPJVXTTUFAA-UHFFFAOYSA-N | |
Source | PubChem | |
URL | https://pubchem.ncbi.nlm.nih.gov | |
Description | Data deposited in or computed by PubChem | |
Canonical SMILES |
CC(=O)NC1=CC=C(C=C1)C(=O)NC2=C(C=CC(=C2)C3=CC=CS3)N | |
Source | PubChem | |
URL | https://pubchem.ncbi.nlm.nih.gov | |
Description | Data deposited in or computed by PubChem | |
Molecular Formula |
C19H17N3O2S | |
Source | PubChem | |
URL | https://pubchem.ncbi.nlm.nih.gov | |
Description | Data deposited in or computed by PubChem | |
Molecular Weight |
351.4 g/mol | |
Source | PubChem | |
URL | https://pubchem.ncbi.nlm.nih.gov | |
Description | Data deposited in or computed by PubChem | |
Product Name |
4-acetamido-N-(2-amino-5-thiophen-2-ylphenyl)benzamide |
Retrosynthesis Analysis
AI-Powered Synthesis Planning: Our tool employs the Template_relevance Pistachio, Template_relevance Bkms_metabolic, Template_relevance Pistachio_ringbreaker, Template_relevance Reaxys, Template_relevance Reaxys_biocatalysis model, leveraging a vast database of chemical reactions to predict feasible synthetic routes.
One-Step Synthesis Focus: Specifically designed for one-step synthesis, it provides concise and direct routes for your target compounds, streamlining the synthesis process.
Accurate Predictions: Utilizing the extensive PISTACHIO, BKMS_METABOLIC, PISTACHIO_RINGBREAKER, REAXYS, REAXYS_BIOCATALYSIS database, our tool offers high-accuracy predictions, reflecting the latest in chemical research and data.
Strategy Settings
Precursor scoring | Relevance Heuristic |
---|---|
Min. plausibility | 0.01 |
Model | Template_relevance |
Template Set | Pistachio/Bkms_metabolic/Pistachio_ringbreaker/Reaxys/Reaxys_biocatalysis |
Top-N result to add to graph | 6 |
Feasible Synthetic Routes
Q & A
Q1: The research paper mentions that Merck60, in combination with Tazemetostat, effectively suppressed cell growth in G401 kidney cancer cells. What is the scientific basis for combining a HDAC1/2 inhibitor with an EZH2 inhibitor in the context of Wilms tumor?
A1: The rationale for combining Merck60 (HDAC1/2 inhibitor) and Tazemetostat (EZH2 inhibitor) stems from the study's findings that both HDAC1/2 and EZH2 are overactive in Wilms tumor cells []. HDAC1/2, by upregulating SIX1/2, and EZH2, by promoting nephron progenitor cell proliferation, contribute to the uncontrolled growth characteristic of this cancer []. By inhibiting both these pathways simultaneously, the researchers aimed to achieve a synergistic effect, effectively disrupting the tumor cells' ability to proliferate. This approach aligns with the growing body of evidence suggesting that combined inhibition of HDACs and EZH2 can be a potent strategy for treating various cancers [].
Haftungsausschluss und Informationen zu In-Vitro-Forschungsprodukten
Bitte beachten Sie, dass alle Artikel und Produktinformationen, die auf BenchChem präsentiert werden, ausschließlich zu Informationszwecken bestimmt sind. Die auf BenchChem zum Kauf angebotenen Produkte sind speziell für In-vitro-Studien konzipiert, die außerhalb lebender Organismen durchgeführt werden. In-vitro-Studien, abgeleitet von dem lateinischen Begriff "in Glas", beinhalten Experimente, die in kontrollierten Laborumgebungen unter Verwendung von Zellen oder Geweben durchgeführt werden. Es ist wichtig zu beachten, dass diese Produkte nicht als Arzneimittel oder Medikamente eingestuft sind und keine Zulassung der FDA für die Vorbeugung, Behandlung oder Heilung von medizinischen Zuständen, Beschwerden oder Krankheiten erhalten haben. Wir müssen betonen, dass jede Form der körperlichen Einführung dieser Produkte in Menschen oder Tiere gesetzlich strikt untersagt ist. Es ist unerlässlich, sich an diese Richtlinien zu halten, um die Einhaltung rechtlicher und ethischer Standards in Forschung und Experiment zu gewährleisten.