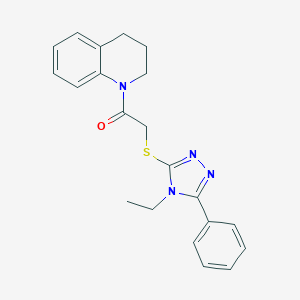
1-(3,4-Dihydroquinolin-1(2H)-yl)-2-((4-ethyl-5-phenyl-4H-1,2,4-triazol-3-yl)thio)ethan-1-one
- Klicken Sie auf QUICK INQUIRY, um ein Angebot von unserem Expertenteam zu erhalten.
- Mit qualitativ hochwertigen Produkten zu einem WETTBEWERBSFÄHIGEN Preis können Sie sich mehr auf Ihre Forschung konzentrieren.
Übersicht
Beschreibung
Vorbereitungsmethoden
Die Synthese von WAY-355344 umfasst mehrere Schritte, die in der Regel mit der Herstellung der Chinolin- und Triazol-Zwischenprodukte beginnen. Die Reaktionsbedingungen erfordern häufig spezielle Reagenzien und Katalysatoren, um die korrekte Bildung des gewünschten Produkts zu gewährleisten. Industrielle Produktionsverfahren können die Skalierung dieser Syntheserouten beinhalten, wobei die Reaktionsbedingungen streng kontrolliert werden, um eine hohe Ausbeute und Reinheit zu gewährleisten .
Chemische Reaktionsanalyse
WAY-355344 unterliegt verschiedenen Arten von chemischen Reaktionen, darunter:
Oxidation: Diese Reaktion kann durch Oxidationsmittel wie Kaliumpermanganat oder Chromtrioxid erleichtert werden.
Reduktion: Häufige Reduktionsmittel wie Lithiumaluminiumhydrid oder Natriumborhydrid können verwendet werden.
Substitution: Nucleophile Substitutionsreaktionen können auftreten, insbesondere an den Chinolin- und Triazol-Moietäten, unter Verwendung von Reagenzien wie Natriummethoxid oder Kalium-tert-butoxid.
Die Hauptprodukte, die aus diesen Reaktionen gebildet werden, hängen von den spezifischen Bedingungen und Reagenzien ab, die verwendet werden .
Wissenschaftliche Forschungsanwendungen
Chemie: Es dient als Modellverbindung für die Untersuchung komplexer organischer Reaktionen und Mechanismen.
Biologie: Forscher untersuchen seine Wechselwirkungen mit biologischen Molekülen, um sein Potenzial als Therapeutikum zu verstehen.
Medizin: WAY-355344 wird auf seine potenzielle Verwendung bei der Behandlung bestimmter Erkrankungen untersucht, insbesondere solcher, die das zentrale Nervensystem betreffen.
Wirkmechanismus
Der Wirkmechanismus von WAY-355344 beinhaltet seine Wechselwirkung mit spezifischen molekularen Zielstrukturen, wie z. B. Enzymen oder Rezeptoren. Es ist bekannt, die Aktivität der 11b-Hydroxysteroid-Dehydrogenase vom Typ 1 zu hemmen, die eine Rolle beim Metabolismus von Glukokortikoiden spielt. Diese Hemmung kann zu verschiedenen physiologischen Effekten führen, was es zu einer interessanten Verbindung für therapeutische Anwendungen macht .
Analyse Chemischer Reaktionen
WAY-355344 undergoes several types of chemical reactions, including:
Oxidation: This reaction can be facilitated by oxidizing agents such as potassium permanganate or chromium trioxide.
Reduction: Common reducing agents like lithium aluminum hydride or sodium borohydride can be used.
Substitution: Nucleophilic substitution reactions can occur, especially at the quinoline and triazole moieties, using reagents like sodium methoxide or potassium tert-butoxide.
The major products formed from these reactions depend on the specific conditions and reagents used .
Wissenschaftliche Forschungsanwendungen
Chemistry: It serves as a model compound for studying complex organic reactions and mechanisms.
Biology: Researchers investigate its interactions with biological molecules to understand its potential as a therapeutic agent.
Medicine: WAY-355344 is explored for its potential use in treating certain medical conditions, particularly those involving the central nervous system.
Wirkmechanismus
The mechanism of action of WAY-355344 involves its interaction with specific molecular targets, such as enzymes or receptors. It is known to inhibit the activity of 11b-hydroxysteroid dehydrogenase type 1, which plays a role in the metabolism of glucocorticoids. This inhibition can lead to various physiological effects, making it a compound of interest for therapeutic applications .
Vergleich Mit ähnlichen Verbindungen
WAY-355344 kann mit anderen Verbindungen verglichen werden, die ähnliche Strukturen oder Funktionen aufweisen. Einige dieser ähnlichen Verbindungen umfassen:
Ethanon, 1-(3,4-Dihydro-1(2H)-chinolinyl)-2-[(4-ethyl-5-phenyl-4H-1,2,4-triazol-3-yl)thio]-: Diese Verbindung teilt eine ähnliche Kernstruktur, kann aber unterschiedliche Substituenten aufweisen, die ihre chemischen Eigenschaften verändern.
Andere 11b-Hydroxysteroid-Dehydrogenase-Inhibitoren: Diese Verbindungen können unterschiedliche chemische Strukturen haben, teilen sich aber dasselbe Zielenzym, wodurch ein Vergleich hinsichtlich Wirksamkeit und Spezifität möglich wird.
WAY-355344 zeichnet sich durch seine einzigartige Kombination von strukturellen Merkmalen und seine spezifische inhibitorische Wirkung auf die 11b-Hydroxysteroid-Dehydrogenase vom Typ 1 aus, was in bestimmten therapeutischen Kontexten Vorteile bieten kann.
Eigenschaften
Molekularformel |
C21H22N4OS |
---|---|
Molekulargewicht |
378.5 g/mol |
IUPAC-Name |
1-(3,4-dihydro-2H-quinolin-1-yl)-2-[(4-ethyl-5-phenyl-1,2,4-triazol-3-yl)sulfanyl]ethanone |
InChI |
InChI=1S/C21H22N4OS/c1-2-24-20(17-10-4-3-5-11-17)22-23-21(24)27-15-19(26)25-14-8-12-16-9-6-7-13-18(16)25/h3-7,9-11,13H,2,8,12,14-15H2,1H3 |
InChI-Schlüssel |
AHXYHSZRKIFPJS-UHFFFAOYSA-N |
SMILES |
CCN1C(=NN=C1SCC(=O)N2CCCC3=CC=CC=C32)C4=CC=CC=C4 |
Kanonische SMILES |
CCN1C(=NN=C1SCC(=O)N2CCCC3=CC=CC=C32)C4=CC=CC=C4 |
Herkunft des Produkts |
United States |
Haftungsausschluss und Informationen zu In-Vitro-Forschungsprodukten
Bitte beachten Sie, dass alle Artikel und Produktinformationen, die auf BenchChem präsentiert werden, ausschließlich zu Informationszwecken bestimmt sind. Die auf BenchChem zum Kauf angebotenen Produkte sind speziell für In-vitro-Studien konzipiert, die außerhalb lebender Organismen durchgeführt werden. In-vitro-Studien, abgeleitet von dem lateinischen Begriff "in Glas", beinhalten Experimente, die in kontrollierten Laborumgebungen unter Verwendung von Zellen oder Geweben durchgeführt werden. Es ist wichtig zu beachten, dass diese Produkte nicht als Arzneimittel oder Medikamente eingestuft sind und keine Zulassung der FDA für die Vorbeugung, Behandlung oder Heilung von medizinischen Zuständen, Beschwerden oder Krankheiten erhalten haben. Wir müssen betonen, dass jede Form der körperlichen Einführung dieser Produkte in Menschen oder Tiere gesetzlich strikt untersagt ist. Es ist unerlässlich, sich an diese Richtlinien zu halten, um die Einhaltung rechtlicher und ethischer Standards in Forschung und Experiment zu gewährleisten.