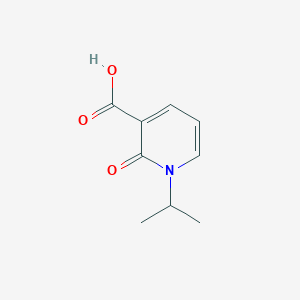
2-Oxo-1-(propan-2-yl)-1,2-dihydropyridine-3-carboxylic acid
- Klicken Sie auf QUICK INQUIRY, um ein Angebot von unserem Expertenteam zu erhalten.
- Mit qualitativ hochwertigen Produkten zu einem WETTBEWERBSFÄHIGEN Preis können Sie sich mehr auf Ihre Forschung konzentrieren.
Übersicht
Beschreibung
Imidazole is a five-membered heterocyclic moiety that possesses three carbon, two nitrogen, four hydrogen atoms, and two double bonds . It is also known as 1, 3-diazole . It contains two nitrogen atoms, in which one nitrogen bear a hydrogen atom, and the other is called pyrrole type nitrogen .
Synthesis Analysis
Pyrrolopyrazine derivatives have various synthetic routes, including cyclization, ring annulation, cycloaddition, direct C-H arylation, and other methods .
Molecular Structure Analysis
Imidazole is a five-membered heterocyclic moiety that possesses three carbon, two nitrogen, four hydrogen atoms, and two double bonds . It is also known as 1, 3-diazole . It contains two nitrogen atoms, in which one nitrogen bear a hydrogen atom, and the other is called pyrrole type nitrogen .
Chemical Reactions Analysis
Pyrrolopyrazine derivatives, including cyclization, ring annulation, cycloaddition, direct C-H arylation, and other methods .
Physical and Chemical Properties Analysis
Imidazole is a white or colorless solid that is highly soluble in water and other polar solvents . Due to the presence of a positive charge on either of two nitrogen atom, it shows two equivalent tautomeric forms .
Wissenschaftliche Forschungsanwendungen
Directed Regulation of Multienzyme Complexes
2-Oxo acid dehydrogenase complexes play critical roles in metabolic pathways, integrating sugar and amino acid degradation. Synthetic analogs of 2-oxo acids, including phosphonate and phosphinate analogs, have been used as selective inhibitors to study these complexes' function in different biological systems. This research highlights the potential of synthetic inhibitors in understanding and modulating metabolism, with implications for systems biology, metabolic engineering, and medicine (Artiukhov, Graf, & Bunik, 2016).
Synthetic Strategies and Pharmacology of Cyanopyridine Derivatives
The 2-oxo-3-cyanopyridine scaffold, structurally related to the query compound, is notable for its wide range of biological activities, including anticancer, antibacterial, and antifungal properties. This review focuses on the synthesis and pharmacological applications of 2-oxo-3-cyanopyridine derivatives, underscoring the scaffold's importance in medicinal chemistry (Ghosh et al., 2015).
Reactive Extraction of Carboxylic Acids
The study of reactive extraction using organic solvents and supercritical fluids offers a glimpse into the separation technologies relevant to carboxylic acids, a functional group present in the compound of interest. This research outlines efficient methods for carboxylic acid separation, highlighting the environmentally benign and recoverable characteristics of supercritical CO2 as a solvent (Djas & Henczka, 2018).
Understanding Biocatalyst Inhibition by Carboxylic Acids
Carboxylic acids, including those structurally related to the query compound, are explored for their inhibitory effects on microbial biocatalysts. This review emphasizes strategies to engineer microbial strains for enhanced tolerance and industrial performance, relevant to biorenewable chemical production (Jarboe, Royce, & Liu, 2013).
Applications in Peptide Studies
The use of spin labels, particularly TOAC, in peptide research provides insight into the conformational and dynamic aspects of peptides. Given the structural similarities of TOAC to pyridine carboxylic acids, this research might suggest potential applications of the query compound in studying peptide structure and interactions (Schreier et al., 2012).
Wirkmechanismus
Target of Action
The primary target of 2-Oxo-1-(propan-2-yl)-1,2-dihydropyridine-3-carboxylic acid is the 5-hydroxytryptamine receptor 4 (5-HT4 receptor) . This receptor is a part of the serotonin receptor family that binds serotonin, a neurotransmitter that regulates various functions such as mood, appetite, and sleep .
Mode of Action
This compound acts as a potent, highly selective agonist with high intrinsic activity at the 5-HT4 receptor . Relative to other 5-HT receptor types, it is > 500-fold selective for binding to the human 5-HT4 receptor . This high degree of selectivity provides the potential for it to be a better and safer medicine for the treatment of patients with severe constipation and possibly constipation predominant irritable bowel syndrome .
Biochemical Pathways
The compound’s interaction with the 5-HT4 receptor triggers a cascade of biochemical reactions. It’s known that 5-HT4 receptor activation typically leads to the production of cyclic AMP (cAMP), which can have various downstream effects depending on the cell type .
Result of Action
The activation of the 5-HT4 receptor by this compound can lead to various molecular and cellular effects. For instance, it can stimulate the release of additional neurotransmitters, modulate the activity of certain ion channels, and alter cellular excitability . These changes can ultimately influence physiological processes such as gastrointestinal motility, which is why the compound may be effective in treating conditions like constipation .
Safety and Hazards
Eigenschaften
IUPAC Name |
2-oxo-1-propan-2-ylpyridine-3-carboxylic acid |
Source
|
---|---|---|
Source | PubChem | |
URL | https://pubchem.ncbi.nlm.nih.gov | |
Description | Data deposited in or computed by PubChem | |
InChI |
InChI=1S/C9H11NO3/c1-6(2)10-5-3-4-7(8(10)11)9(12)13/h3-6H,1-2H3,(H,12,13) |
Source
|
Source | PubChem | |
URL | https://pubchem.ncbi.nlm.nih.gov | |
Description | Data deposited in or computed by PubChem | |
InChI Key |
WMHUUEGGTAJRCB-UHFFFAOYSA-N |
Source
|
Source | PubChem | |
URL | https://pubchem.ncbi.nlm.nih.gov | |
Description | Data deposited in or computed by PubChem | |
Canonical SMILES |
CC(C)N1C=CC=C(C1=O)C(=O)O |
Source
|
Source | PubChem | |
URL | https://pubchem.ncbi.nlm.nih.gov | |
Description | Data deposited in or computed by PubChem | |
Molecular Formula |
C9H11NO3 |
Source
|
Source | PubChem | |
URL | https://pubchem.ncbi.nlm.nih.gov | |
Description | Data deposited in or computed by PubChem | |
Molecular Weight |
181.19 g/mol |
Source
|
Source | PubChem | |
URL | https://pubchem.ncbi.nlm.nih.gov | |
Description | Data deposited in or computed by PubChem | |
Haftungsausschluss und Informationen zu In-Vitro-Forschungsprodukten
Bitte beachten Sie, dass alle Artikel und Produktinformationen, die auf BenchChem präsentiert werden, ausschließlich zu Informationszwecken bestimmt sind. Die auf BenchChem zum Kauf angebotenen Produkte sind speziell für In-vitro-Studien konzipiert, die außerhalb lebender Organismen durchgeführt werden. In-vitro-Studien, abgeleitet von dem lateinischen Begriff "in Glas", beinhalten Experimente, die in kontrollierten Laborumgebungen unter Verwendung von Zellen oder Geweben durchgeführt werden. Es ist wichtig zu beachten, dass diese Produkte nicht als Arzneimittel oder Medikamente eingestuft sind und keine Zulassung der FDA für die Vorbeugung, Behandlung oder Heilung von medizinischen Zuständen, Beschwerden oder Krankheiten erhalten haben. Wir müssen betonen, dass jede Form der körperlichen Einführung dieser Produkte in Menschen oder Tiere gesetzlich strikt untersagt ist. Es ist unerlässlich, sich an diese Richtlinien zu halten, um die Einhaltung rechtlicher und ethischer Standards in Forschung und Experiment zu gewährleisten.