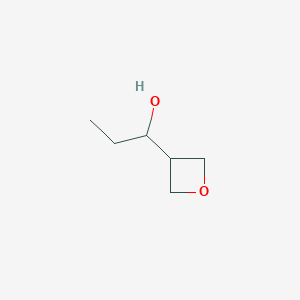
1-(Oxetan-3-yl)propan-1-ol
Übersicht
Beschreibung
1-(Oxetan-3-yl)propan-1-ol is an organic compound that features an oxetane ring, a four-membered cyclic ether, attached to a propanol group
Wirkmechanismus
Target of Action
Oxetane derivatives have been employed to improve drugs’ physicochemical properties . They have been used in the pharmaceutical industry, suggesting that they may interact with various biological targets depending on the specific drug molecule they are incorporated into .
Mode of Action
Oxetanes are known to be more metabolically stable and lipophilicity neutral . As an electron-withdrawing group, it reduces the basicity of its adjacent nitrogen atom, which may subtly modulate the basicity and potentially lower the drug’s overall lipophilicity .
Biochemical Pathways
Oxetanes have been used in medicinal chemistry, suggesting that they may influence a variety of biochemical pathways depending on the specific drug molecule they are part of .
Pharmacokinetics
Oxetanes are known to be more metabolically stable and lipophilicity neutral, which could impact their bioavailability .
Result of Action
The use of oxetanes in drug discovery suggests that they may have a variety of effects depending on the specific drug molecule they are incorporated into .
Action Environment
The stability and efficacy of oxetanes in drug molecules may be influenced by factors such as temperature, ph, and the presence of other substances .
Biochemische Analyse
Biochemical Properties
1-(Oxetan-3-yl)propan-1-ol plays a significant role in various biochemical reactions. It interacts with several enzymes and proteins, influencing their activity and function. For instance, it has been observed to interact with cytochrome P450 enzymes, which are crucial for the metabolism of many drugs and endogenous compounds . The interaction between this compound and these enzymes can lead to either inhibition or activation, depending on the specific enzyme and the context of the reaction. Additionally, this compound can form hydrogen bonds with proteins, affecting their conformation and stability.
Cellular Effects
The effects of this compound on various cell types and cellular processes are profound. It has been shown to influence cell signaling pathways, particularly those involved in oxidative stress and inflammation. By modulating these pathways, this compound can alter gene expression and cellular metabolism. For example, it can upregulate the expression of antioxidant genes, thereby enhancing the cell’s ability to combat oxidative damage. Furthermore, this compound can affect cellular metabolism by altering the activity of key metabolic enzymes.
Molecular Mechanism
At the molecular level, this compound exerts its effects through several mechanisms. One primary mechanism is its ability to bind to specific biomolecules, such as enzymes and receptors . This binding can lead to changes in the activity of these biomolecules, either inhibiting or activating their function. For instance, this compound can inhibit the activity of certain enzymes by occupying their active sites, preventing substrate binding. Additionally, it can activate other enzymes by inducing conformational changes that enhance their catalytic activity. Changes in gene expression are also a result of its interaction with transcription factors and other regulatory proteins.
Temporal Effects in Laboratory Settings
The effects of this compound can change over time in laboratory settings. This compound is relatively stable under standard laboratory conditions, but it can degrade over extended periods or under harsh conditions . Long-term studies have shown that prolonged exposure to this compound can lead to adaptive changes in cellular function, such as increased expression of detoxifying enzymes. These changes can influence the overall cellular response to the compound, potentially leading to altered efficacy or toxicity.
Dosage Effects in Animal Models
In animal models, the effects of this compound vary with different dosages. At low doses, it can have beneficial effects, such as enhancing antioxidant defenses and reducing inflammation . At higher doses, it can exhibit toxic effects, including liver damage and oxidative stress. Threshold effects have been observed, where a specific dose range produces maximal therapeutic benefits without significant toxicity. Beyond this range, the adverse effects outweigh the benefits, highlighting the importance of dose optimization in therapeutic applications.
Metabolic Pathways
This compound is involved in several metabolic pathways. It is primarily metabolized by cytochrome P450 enzymes, which convert it into various metabolites . These metabolites can then enter different metabolic pathways, influencing metabolic flux and metabolite levels. For example, some metabolites may be further processed by conjugation reactions, leading to their excretion from the body. The interaction of this compound with metabolic enzymes can also affect the levels of other metabolites, potentially leading to metabolic imbalances.
Transport and Distribution
Within cells and tissues, this compound is transported and distributed through various mechanisms. It can diffuse across cell membranes due to its relatively small size and moderate polarity . Additionally, it may interact with specific transporters or binding proteins that facilitate its movement within the cell. The distribution of this compound can affect its localization and accumulation, influencing its overall activity and function.
Subcellular Localization
The subcellular localization of this compound is crucial for its activity. It can be found in various cellular compartments, including the cytoplasm, mitochondria, and nucleus . The localization is often determined by targeting signals or post-translational modifications that direct the compound to specific organelles. For instance, the presence of specific functional groups on this compound can facilitate its transport into the mitochondria, where it can influence mitochondrial function and energy metabolism.
Vorbereitungsmethoden
Synthetic Routes and Reaction Conditions
1-(Oxetan-3-yl)propan-1-ol can be synthesized through several methods. One common approach involves the cyclization of 3-chloropropyl acetate with potassium hydroxide at elevated temperatures . Another method includes the Paternò–Büchi reaction, which forms the oxetane ring through a [2+2] cycloaddition of an alkene with a carbonyl compound . Additionally, diol cyclization and decarboxylation of six-membered cyclic carbonates are also viable routes .
Industrial Production Methods
Industrial production of this compound typically involves large-scale synthesis using optimized reaction conditions to maximize yield and purity. The choice of method depends on factors such as cost, availability of starting materials, and desired product specifications.
Analyse Chemischer Reaktionen
Types of Reactions
1-(Oxetan-3-yl)propan-1-ol undergoes various chemical reactions, including:
Oxidation: The hydroxyl group can be oxidized to form corresponding aldehydes or carboxylic acids.
Reduction: The compound can be reduced to form different alcohol derivatives.
Substitution: The oxetane ring can undergo nucleophilic substitution reactions, leading to ring-opening and formation of new compounds.
Common Reagents and Conditions
Oxidation: Common oxidizing agents include potassium permanganate (KMnO₄) and chromium trioxide (CrO₃).
Reduction: Reducing agents such as lithium aluminum hydride (LiAlH₄) are often used.
Substitution: Nucleophiles like amines or thiols can be used under basic conditions to open the oxetane ring.
Major Products Formed
The major products formed from these reactions depend on the specific reagents and conditions used. For example, oxidation can yield aldehydes or carboxylic acids, while substitution reactions can produce a variety of ring-opened derivatives .
Wissenschaftliche Forschungsanwendungen
1-(Oxetan-3-yl)propan-1-ol has several scientific research applications:
Vergleich Mit ähnlichen Verbindungen
Similar Compounds
1,3-Propylene oxide: Another oxetane derivative with similar reactivity but different applications.
3-Methyloxetane: A methyl-substituted oxetane with distinct properties and uses.
3,3-Bis(chloromethyl)oxetane: A chlorinated oxetane used in specialized chemical processes.
Uniqueness
1-(Oxetan-3-yl)propan-1-ol is unique due to its combination of the oxetane ring and propanol group, which imparts specific reactivity and potential for diverse applications. Its ability to undergo various chemical transformations and its role in synthesizing complex molecules make it a valuable compound in research and industry .
Eigenschaften
IUPAC Name |
1-(oxetan-3-yl)propan-1-ol | |
---|---|---|
Details | Computed by LexiChem 2.6.6 (PubChem release 2019.06.18) | |
Source | PubChem | |
URL | https://pubchem.ncbi.nlm.nih.gov | |
Description | Data deposited in or computed by PubChem | |
InChI |
InChI=1S/C6H12O2/c1-2-6(7)5-3-8-4-5/h5-7H,2-4H2,1H3 | |
Details | Computed by InChI 1.0.5 (PubChem release 2019.06.18) | |
Source | PubChem | |
URL | https://pubchem.ncbi.nlm.nih.gov | |
Description | Data deposited in or computed by PubChem | |
InChI Key |
HACRLPBEGSHDAA-UHFFFAOYSA-N | |
Details | Computed by InChI 1.0.5 (PubChem release 2019.06.18) | |
Source | PubChem | |
URL | https://pubchem.ncbi.nlm.nih.gov | |
Description | Data deposited in or computed by PubChem | |
Canonical SMILES |
CCC(C1COC1)O | |
Details | Computed by OEChem 2.1.5 (PubChem release 2019.06.18) | |
Source | PubChem | |
URL | https://pubchem.ncbi.nlm.nih.gov | |
Description | Data deposited in or computed by PubChem | |
Molecular Formula |
C6H12O2 | |
Details | Computed by PubChem 2.1 (PubChem release 2019.06.18) | |
Source | PubChem | |
URL | https://pubchem.ncbi.nlm.nih.gov | |
Description | Data deposited in or computed by PubChem | |
Molecular Weight |
116.16 g/mol | |
Details | Computed by PubChem 2.1 (PubChem release 2021.05.07) | |
Source | PubChem | |
URL | https://pubchem.ncbi.nlm.nih.gov | |
Description | Data deposited in or computed by PubChem | |
Retrosynthesis Analysis
AI-Powered Synthesis Planning: Our tool employs the Template_relevance Pistachio, Template_relevance Bkms_metabolic, Template_relevance Pistachio_ringbreaker, Template_relevance Reaxys, Template_relevance Reaxys_biocatalysis model, leveraging a vast database of chemical reactions to predict feasible synthetic routes.
One-Step Synthesis Focus: Specifically designed for one-step synthesis, it provides concise and direct routes for your target compounds, streamlining the synthesis process.
Accurate Predictions: Utilizing the extensive PISTACHIO, BKMS_METABOLIC, PISTACHIO_RINGBREAKER, REAXYS, REAXYS_BIOCATALYSIS database, our tool offers high-accuracy predictions, reflecting the latest in chemical research and data.
Strategy Settings
Precursor scoring | Relevance Heuristic |
---|---|
Min. plausibility | 0.01 |
Model | Template_relevance |
Template Set | Pistachio/Bkms_metabolic/Pistachio_ringbreaker/Reaxys/Reaxys_biocatalysis |
Top-N result to add to graph | 6 |
Feasible Synthetic Routes
Haftungsausschluss und Informationen zu In-Vitro-Forschungsprodukten
Bitte beachten Sie, dass alle Artikel und Produktinformationen, die auf BenchChem präsentiert werden, ausschließlich zu Informationszwecken bestimmt sind. Die auf BenchChem zum Kauf angebotenen Produkte sind speziell für In-vitro-Studien konzipiert, die außerhalb lebender Organismen durchgeführt werden. In-vitro-Studien, abgeleitet von dem lateinischen Begriff "in Glas", beinhalten Experimente, die in kontrollierten Laborumgebungen unter Verwendung von Zellen oder Geweben durchgeführt werden. Es ist wichtig zu beachten, dass diese Produkte nicht als Arzneimittel oder Medikamente eingestuft sind und keine Zulassung der FDA für die Vorbeugung, Behandlung oder Heilung von medizinischen Zuständen, Beschwerden oder Krankheiten erhalten haben. Wir müssen betonen, dass jede Form der körperlichen Einführung dieser Produkte in Menschen oder Tiere gesetzlich strikt untersagt ist. Es ist unerlässlich, sich an diese Richtlinien zu halten, um die Einhaltung rechtlicher und ethischer Standards in Forschung und Experiment zu gewährleisten.