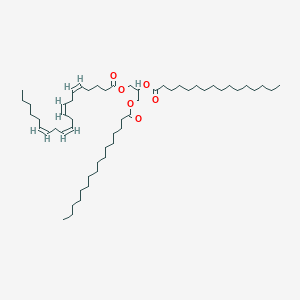
2,3-Di(hexadecanoyloxy)propyl (5Z,8Z,11Z,14Z)-icosa-5,8,11,14-tetraenoate
Übersicht
Beschreibung
Vorbereitungsmethoden
1,2-Dipalmitoyl-3-Arachidonoyl-rac-glycerol can be synthesized through enzymatic methods. One such method involves the use of lipase-catalyzed reactions to incorporate palmitic acid and arachidonic acid into the glycerol backbone . The reaction conditions typically include controlled temperature and pH to optimize the enzyme activity . Industrial production methods may involve similar enzymatic processes but on a larger scale, ensuring high purity and yield of the compound .
Analyse Chemischer Reaktionen
1,2-Dipalmitoyl-3-Arachidonoyl-rac-glycerol undergoes various chemical reactions, including oxidation and hydrolysis . Common reagents used in these reactions include oxidizing agents like potassium permanganate and hydrolyzing agents like sodium hydroxide . The major products formed from these reactions include free fatty acids and glycerol .
Wissenschaftliche Forschungsanwendungen
1,2-Dipalmitoyl-3-Arachidonoyl-rac-glycerol has several scientific research applications. In chemistry, it is used as a model compound to study lipid behavior and interactions . In biology, it is studied for its role in cell membrane structure and function . In medicine, it is investigated for its potential therapeutic effects, particularly in relation to inflammation and metabolic disorders . In industry, it is used in the formulation of various lipid-based products .
Wirkmechanismus
The mechanism of action of 1,2-Dipalmitoyl-3-Arachidonoyl-rac-glycerol involves its incorporation into cell membranes, where it influences membrane fluidity and signaling pathways . The molecular targets include membrane-bound enzymes and receptors that are involved in lipid metabolism and inflammatory responses .
Vergleich Mit ähnlichen Verbindungen
1,2-Dipalmitoyl-3-Arachidonoyl-rac-glycerol can be compared with other triacylglycerols such as 1,2-Dipalmitoyl-3-Oleoyl-rac-glycerol and 1,2-Dipalmitoyl-3-Linoleoyl-rac-glycerol . These compounds differ in the types of fatty acids they contain, which affects their physical and chemical properties . The presence of arachidonic acid in 1,2-Dipalmitoyl-3-Arachidonoyl-rac-glycerol makes it unique due to its role in inflammatory processes and cell signaling .
Eigenschaften
IUPAC Name |
2,3-di(hexadecanoyloxy)propyl (5Z,8Z,11Z,14Z)-icosa-5,8,11,14-tetraenoate | |
---|---|---|
Details | Computed by LexiChem 2.6.6 (PubChem release 2019.06.18) | |
Source | PubChem | |
URL | https://pubchem.ncbi.nlm.nih.gov | |
Description | Data deposited in or computed by PubChem | |
InChI |
InChI=1S/C55H98O6/c1-4-7-10-13-16-19-22-25-26-27-28-31-33-36-39-42-45-48-54(57)60-51-52(61-55(58)49-46-43-40-37-34-30-24-21-18-15-12-9-6-3)50-59-53(56)47-44-41-38-35-32-29-23-20-17-14-11-8-5-2/h16,19,25-26,28,31,36,39,52H,4-15,17-18,20-24,27,29-30,32-35,37-38,40-51H2,1-3H3/b19-16-,26-25-,31-28-,39-36- | |
Details | Computed by InChI 1.0.5 (PubChem release 2019.06.18) | |
Source | PubChem | |
URL | https://pubchem.ncbi.nlm.nih.gov | |
Description | Data deposited in or computed by PubChem | |
InChI Key |
FTMDHBKIGZUTJX-MFRLHHNRSA-N | |
Details | Computed by InChI 1.0.5 (PubChem release 2019.06.18) | |
Source | PubChem | |
URL | https://pubchem.ncbi.nlm.nih.gov | |
Description | Data deposited in or computed by PubChem | |
Canonical SMILES |
CCCCCCCCCCCCCCCC(=O)OCC(COC(=O)CCCC=CCC=CCC=CCC=CCCCCC)OC(=O)CCCCCCCCCCCCCCC | |
Details | Computed by OEChem 2.1.5 (PubChem release 2019.06.18) | |
Source | PubChem | |
URL | https://pubchem.ncbi.nlm.nih.gov | |
Description | Data deposited in or computed by PubChem | |
Isomeric SMILES |
CCCCCCCCCCCCCCCC(=O)OCC(COC(=O)CCC/C=C\C/C=C\C/C=C\C/C=C\CCCCC)OC(=O)CCCCCCCCCCCCCCC | |
Details | Computed by OEChem 2.1.5 (PubChem release 2019.06.18) | |
Source | PubChem | |
URL | https://pubchem.ncbi.nlm.nih.gov | |
Description | Data deposited in or computed by PubChem | |
Molecular Formula |
C55H98O6 | |
Details | Computed by PubChem 2.1 (PubChem release 2019.06.18) | |
Source | PubChem | |
URL | https://pubchem.ncbi.nlm.nih.gov | |
Description | Data deposited in or computed by PubChem | |
Molecular Weight |
855.4 g/mol | |
Details | Computed by PubChem 2.1 (PubChem release 2021.05.07) | |
Source | PubChem | |
URL | https://pubchem.ncbi.nlm.nih.gov | |
Description | Data deposited in or computed by PubChem | |
Retrosynthesis Analysis
AI-Powered Synthesis Planning: Our tool employs the Template_relevance Pistachio, Template_relevance Bkms_metabolic, Template_relevance Pistachio_ringbreaker, Template_relevance Reaxys, Template_relevance Reaxys_biocatalysis model, leveraging a vast database of chemical reactions to predict feasible synthetic routes.
One-Step Synthesis Focus: Specifically designed for one-step synthesis, it provides concise and direct routes for your target compounds, streamlining the synthesis process.
Accurate Predictions: Utilizing the extensive PISTACHIO, BKMS_METABOLIC, PISTACHIO_RINGBREAKER, REAXYS, REAXYS_BIOCATALYSIS database, our tool offers high-accuracy predictions, reflecting the latest in chemical research and data.
Strategy Settings
Precursor scoring | Relevance Heuristic |
---|---|
Min. plausibility | 0.01 |
Model | Template_relevance |
Template Set | Pistachio/Bkms_metabolic/Pistachio_ringbreaker/Reaxys/Reaxys_biocatalysis |
Top-N result to add to graph | 6 |
Feasible Synthetic Routes
Haftungsausschluss und Informationen zu In-Vitro-Forschungsprodukten
Bitte beachten Sie, dass alle Artikel und Produktinformationen, die auf BenchChem präsentiert werden, ausschließlich zu Informationszwecken bestimmt sind. Die auf BenchChem zum Kauf angebotenen Produkte sind speziell für In-vitro-Studien konzipiert, die außerhalb lebender Organismen durchgeführt werden. In-vitro-Studien, abgeleitet von dem lateinischen Begriff "in Glas", beinhalten Experimente, die in kontrollierten Laborumgebungen unter Verwendung von Zellen oder Geweben durchgeführt werden. Es ist wichtig zu beachten, dass diese Produkte nicht als Arzneimittel oder Medikamente eingestuft sind und keine Zulassung der FDA für die Vorbeugung, Behandlung oder Heilung von medizinischen Zuständen, Beschwerden oder Krankheiten erhalten haben. Wir müssen betonen, dass jede Form der körperlichen Einführung dieser Produkte in Menschen oder Tiere gesetzlich strikt untersagt ist. Es ist unerlässlich, sich an diese Richtlinien zu halten, um die Einhaltung rechtlicher und ethischer Standards in Forschung und Experiment zu gewährleisten.