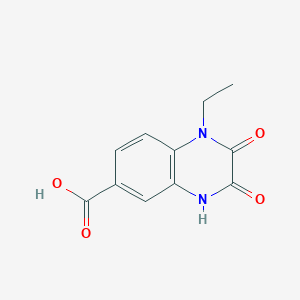
1-Ethyl-2,3-dioxo-1,2,3,4-tetrahydroquinoxaline-6-carboxylic acid
Übersicht
Beschreibung
1-Ethyl-2,3-dioxo-1,2,3,4-tetrahydroquinoxaline-6-carboxylic acid (CAS: 744227-07-4) is a quinoxaline derivative featuring an ethyl group at the N1 position, two ketone groups at C2 and C3, and a carboxylic acid substituent at C6 (Figure 1). Its molecular formula is C₁₁H₁₀N₂O₅, with a molecular weight of 250.21 g/mol. The compound is commercially available from suppliers such as Combi-Blocks (ST-4810) and ABChem (AB9262), with prices ranging from €463 to €1,855 per 250–2500 mg .
Structurally, it belongs to the tetrahydroquinoxaline family, which is known for diverse bioactivities. Evidence suggests that N-substituted 3-oxo-tetrahydroquinoxaline-6-carboxylic acid derivatives inhibit tubulin polymerization, a mechanism leveraged in anticancer drug development .
Vorbereitungsmethoden
Synthetic Routes and Reaction Conditions: The synthesis of 1-Ethyl-2,3-dioxo-1,2,3,4-tetrahydroquinoxaline-6-carboxylic acid typically involves the condensation of ethyl anthranilate with 1,2-diketones under acidic conditions. The reaction proceeds through the formation of an intermediate quinoxaline derivative, which is then oxidized to yield the final product.
Industrial Production Methods: In an industrial setting, the compound is synthesized using large-scale reactors with precise control over temperature and pH. The use of catalysts such as sulfuric acid or polyphosphoric acid can enhance the reaction efficiency and yield. Purification steps, including recrystallization and chromatography, are employed to obtain the compound in high purity.
Analyse Chemischer Reaktionen
Types of Reactions: 1-Ethyl-2,3-dioxo-1,2,3,4-tetrahydroquinoxaline-6-carboxylic acid undergoes various chemical reactions, including:
Oxidation: The compound can be oxidized to form quinoxaline-2,3-dione derivatives.
Reduction: Reduction reactions can lead to the formation of reduced quinoxaline derivatives.
Substitution: Electrophilic and nucleophilic substitution reactions can occur at different positions on the quinoxaline ring.
Common Reagents and Conditions:
Oxidation: Common oxidizing agents include potassium permanganate, chromic acid, and hydrogen peroxide.
Reduction: Reducing agents such as lithium aluminum hydride (LiAlH4) and sodium borohydride (NaBH4) are used.
Substitution: Reagents like halogens (e.g., chlorine, bromine) and strong bases (e.g., sodium hydroxide) are employed.
Major Products Formed:
Oxidation: Quinoxaline-2,3-dione derivatives.
Reduction: Reduced quinoxaline derivatives.
Substitution: Halogenated or alkylated quinoxaline derivatives.
Wissenschaftliche Forschungsanwendungen
Structural Information
- Molecular Formula : CHNO
- Molecular Weight : 234.21 g/mol
- IUPAC Name : 1-ethyl-2,3-dioxo-1,2,3,4-tetrahydroquinoxaline-6-carboxylic acid
- CAS Number : 744227-07-4
Predicted Collision Cross Section
Adduct | m/z | Predicted CCS (Ų) |
---|---|---|
[M+H]+ | 235.07134 | 148.5 |
[M+Na]+ | 257.05328 | 161.9 |
[M+NH₄]+ | 252.09788 | 154.1 |
[M+K]+ | 273.02722 | 157.3 |
[M-H]− | 233.05678 | 147.6 |
Medicinal Chemistry
This compound has been investigated for its potential as a therapeutic agent due to its structural resemblance to bioactive compounds. Research indicates that derivatives of quinoxaline exhibit antimicrobial and anticancer properties.
Case Study: Antimicrobial Activity
A study published in a peer-reviewed journal demonstrated that quinoxaline derivatives showed significant activity against various bacterial strains, suggesting that this compound could be further explored for developing new antibiotics.
Biochemical Applications
This compound has also been utilized in biochemical assays to study enzyme inhibition and protein interactions. The dioxo moiety can interact with various biological targets, making it a candidate for further exploration in drug design.
Case Study: Enzyme Inhibition
Research conducted on the inhibition of specific enzymes involved in metabolic pathways has shown that compounds similar to this compound can effectively inhibit target enzymes like dihydrofolate reductase (DHFR), which is crucial in cancer therapy.
Material Science
The compound's unique chemical structure allows it to be studied as a potential material for organic electronics and photonic devices due to its electronic properties.
Case Study: Organic Photovoltaics
Investigations into the use of quinoxaline derivatives in organic photovoltaic cells have revealed enhanced charge transport properties when incorporated into polymer blends, indicating potential applications in solar energy conversion technologies.
Wirkmechanismus
The mechanism by which 1-Ethyl-2,3-dioxo-1,2,3,4-tetrahydroquinoxaline-6-carboxylic acid exerts its effects involves interaction with specific molecular targets and pathways. The compound may bind to enzymes or receptors, leading to the modulation of biological processes. The exact mechanism can vary depending on the specific application and target.
Vergleich Mit ähnlichen Verbindungen
Comparison with Similar Compounds
Structural and Functional Group Variations
The compound’s activity and physicochemical properties are influenced by its substituents. Below is a comparative analysis with key analogs:
Table 1: Key Structural and Functional Comparisons
Physicochemical and Commercial Considerations
- Solubility: The carboxylic acid group enhances water solubility compared to ester or sulfonamide derivatives, though methyl esters (e.g., methyl 3-oxo-tetrahydroquinoxaline-6-carboxylate) may improve lipid membrane permeability .
- Stereochemistry : The (2R)-2-ethyl-3-oxo analog demonstrates that stereochemical positioning (C2 vs. N1 substitution) alters molecular interactions, though its bioactivity remains unexplored .
- Availability : The target compound is more accessible (priced at €463/250 mg) than specialized analogs like N,N-dibenzyl-sulfonamide derivatives, which require custom synthesis .
Biologische Aktivität
1-Ethyl-2,3-dioxo-1,2,3,4-tetrahydroquinoxaline-6-carboxylic acid (CAS Number: 744227-07-4) is a heterocyclic compound that has garnered attention for its potential biological activities. This compound is part of a larger class of quinoxaline derivatives known for diverse pharmacological properties, including anticancer, antimicrobial, and neuropharmacological effects. This article aims to synthesize findings from various studies to provide a comprehensive overview of the biological activity associated with this compound.
- Molecular Formula : C₁₁H₁₀N₂O₄
- Molecular Weight : 234.21 g/mol
- Structure : The compound features a quinoxaline core with dioxo and carboxylic acid functional groups which are critical for its biological activity.
Anticancer Activity
Research has demonstrated that derivatives of this compound exhibit significant antiproliferative effects against various cancer cell lines. For instance:
- Study Findings : A series of novel N-substituted derivatives were synthesized and evaluated for their biological activities. One derivative (13d) showed potent antiproliferative activity against HeLa (cervical cancer), SMMC-7721 (hepatocellular carcinoma), and K562 (leukemia) cell lines with IC₅₀ values of 0.126 μM, 0.071 μM, and 0.164 μM respectively. Additionally, this compound inhibited tubulin polymerization (IC₅₀ = 3.97 μM), arrested the cell cycle at the G2/M phase, and induced apoptosis .
Cell Line | IC₅₀ (μM) |
---|---|
HeLa | 0.126 |
SMMC-7721 | 0.071 |
K562 | 0.164 |
Neuropharmacological Activity
Quinoxaline derivatives have also been explored for their neuropharmacological effects:
- Anxiolytic Effects : In animal models, certain derivatives exhibited anxiolytic effects comparable to diazepam at specific doses. For example, N,N-dibenzyl-2,3-dioxo-1,2,3,4-tetrahydroquinoxaline-6-sulfonamide showed significant activity at a dose of 2.5 mg/kg .
Antimicrobial Activity
The antimicrobial potential of quinoxaline derivatives has been extensively studied:
- Antifungal Activity : A study indicated that synthesized quinoxaline dione derivatives displayed antifungal activity against various strains such as Candida albicans and Aspergillus species with Minimum Inhibitory Concentration (MIC) values ranging from 12.8 to 15.9 μg/ml .
Microorganism | MIC (μg/ml) |
---|---|
Candida albicans | 12.8 |
Aspergillus fumigatus | 15.9 |
Structure-Activity Relationship (SAR)
The biological activity of quinoxaline derivatives often correlates with their structural features:
- Substituent Influence : The presence of specific functional groups can enhance or diminish the biological efficacy of these compounds. For instance, introducing electron-withdrawing groups at certain positions on the quinoxaline structure may increase antiproliferative activity .
Case Studies
Several case studies illustrate the diverse applications of quinoxaline derivatives:
- PARP Inhibition : Recent studies have shown that certain derivatives act as inhibitors of PARP-1 enzyme with IC₅₀ values in the nanomolar range (e.g., IC₅₀ = 3.05 nM). These findings highlight their potential in cancer therapy by targeting DNA repair mechanisms .
- Tubulin Polymerization : The ability of these compounds to inhibit tubulin polymerization suggests a mechanism similar to that of known chemotherapeutic agents like taxanes and vinca alkaloids .
Q & A
Basic Research Questions
Q. What are the optimal synthetic routes for 1-ethyl-2,3-dioxo-1,2,3,4-tetrahydroquinoxaline-6-carboxylic acid, and how do reaction conditions influence yield and purity?
Methodological Answer: The compound can be synthesized via cyclocondensation of ethylenediamine derivatives with α-keto acids or esters. Key parameters include:
- Temperature control : Elevated temperatures (80–120°C) promote ring closure but may increase side products like decarboxylated derivatives .
- Catalysts : Acidic conditions (e.g., HCl or H₂SO₄) accelerate imine formation, while base catalysts (e.g., NaHCO₃) improve carboxylate stability .
- Purification : Reverse-phase chromatography or recrystallization from ethanol/water mixtures enhances purity, with yields typically ranging from 45–70% depending on substituent steric effects .
Q. What analytical techniques are most effective for characterizing the structural conformation of this compound, especially regarding its stereochemistry?
Methodological Answer:
- X-ray crystallography : Resolves absolute stereochemistry (e.g., the (2R)-configuration confirmed in PDB entry 5GV) and hydrogen-bonding networks critical for biological activity .
- NMR spectroscopy : H and C NMR distinguish between tautomeric forms (e.g., keto-enol equilibria) and verify the ethyl group’s position at C2 .
- Circular Dichroism (CD) : Detects chiral centers in solution phase, complementing crystallographic data .
Advanced Research Questions
Q. How can computational chemistry be integrated into the design of derivatives to predict biological activity and optimize pharmacokinetics?
Methodological Answer:
- Quantum mechanical calculations (DFT) : Predict electronic properties (e.g., HOMO/LUMO energies) to guide substitutions at C6 (carboxylic acid) or C2 (ethyl group) for enhanced target binding .
- Molecular docking : Screen derivatives against enzymes like dihydroorotate dehydrogenase (DHODH), where the quinoxaline scaffold shows affinity for the flavin-binding pocket .
- ADMET prediction : Tools like SwissADME assess logP, solubility, and metabolic stability, prioritizing derivatives with reduced hepatotoxicity .
Q. How should researchers resolve contradictory data regarding the compound’s biological activity across different studies?
Methodological Answer:
- Meta-analysis : Systematically compare assay conditions (e.g., cell lines, incubation times) that may explain variability. For example, IC₅₀ values in cancer cells vary with glutathione levels, which quench reactive oxygen species (ROS) generated by the compound .
- Dose-response validation : Use orthogonal assays (e.g., enzymatic vs. cell-based) to confirm target specificity. Contradictions in antimicrobial activity may arise from differences in bacterial membrane permeability .
- Statistical DoE (Design of Experiments) : Apply factorial designs to isolate variables (e.g., pH, temperature) affecting bioactivity reproducibility .
Q. What are the key considerations in designing in vitro assays to evaluate the compound’s enzyme inhibition potential?
Methodological Answer:
- Enzyme selection : Prioritize targets with structural homology to known quinoxaline interactors, such as xanthine oxidase or tyrosine kinases .
- Assay conditions :
- pH optimization : Maintain physiological pH (7.4) to mimic in vivo environments, but test acidic pH (5.5–6.5) for lysosomal targeting .
- Substrate competition : Use kinetic assays (e.g., spectrophotometric monitoring of NADH depletion) to distinguish competitive vs. non-competitive inhibition .
- Control experiments : Include positive controls (e.g., allopurinol for xanthine oxidase) and assess off-target effects via counter-screening .
Eigenschaften
IUPAC Name |
1-ethyl-2,3-dioxo-4H-quinoxaline-6-carboxylic acid | |
---|---|---|
Details | Computed by LexiChem 2.6.6 (PubChem release 2019.06.18) | |
Source | PubChem | |
URL | https://pubchem.ncbi.nlm.nih.gov | |
Description | Data deposited in or computed by PubChem | |
InChI |
InChI=1S/C11H10N2O4/c1-2-13-8-4-3-6(11(16)17)5-7(8)12-9(14)10(13)15/h3-5H,2H2,1H3,(H,12,14)(H,16,17) | |
Details | Computed by InChI 1.0.5 (PubChem release 2019.06.18) | |
Source | PubChem | |
URL | https://pubchem.ncbi.nlm.nih.gov | |
Description | Data deposited in or computed by PubChem | |
InChI Key |
FYFZKHVVUSQPKC-UHFFFAOYSA-N | |
Details | Computed by InChI 1.0.5 (PubChem release 2019.06.18) | |
Source | PubChem | |
URL | https://pubchem.ncbi.nlm.nih.gov | |
Description | Data deposited in or computed by PubChem | |
Canonical SMILES |
CCN1C2=C(C=C(C=C2)C(=O)O)NC(=O)C1=O | |
Details | Computed by OEChem 2.1.5 (PubChem release 2019.06.18) | |
Source | PubChem | |
URL | https://pubchem.ncbi.nlm.nih.gov | |
Description | Data deposited in or computed by PubChem | |
Molecular Formula |
C11H10N2O4 | |
Details | Computed by PubChem 2.1 (PubChem release 2019.06.18) | |
Source | PubChem | |
URL | https://pubchem.ncbi.nlm.nih.gov | |
Description | Data deposited in or computed by PubChem | |
Molecular Weight |
234.21 g/mol | |
Details | Computed by PubChem 2.1 (PubChem release 2021.05.07) | |
Source | PubChem | |
URL | https://pubchem.ncbi.nlm.nih.gov | |
Description | Data deposited in or computed by PubChem | |
Haftungsausschluss und Informationen zu In-Vitro-Forschungsprodukten
Bitte beachten Sie, dass alle Artikel und Produktinformationen, die auf BenchChem präsentiert werden, ausschließlich zu Informationszwecken bestimmt sind. Die auf BenchChem zum Kauf angebotenen Produkte sind speziell für In-vitro-Studien konzipiert, die außerhalb lebender Organismen durchgeführt werden. In-vitro-Studien, abgeleitet von dem lateinischen Begriff "in Glas", beinhalten Experimente, die in kontrollierten Laborumgebungen unter Verwendung von Zellen oder Geweben durchgeführt werden. Es ist wichtig zu beachten, dass diese Produkte nicht als Arzneimittel oder Medikamente eingestuft sind und keine Zulassung der FDA für die Vorbeugung, Behandlung oder Heilung von medizinischen Zuständen, Beschwerden oder Krankheiten erhalten haben. Wir müssen betonen, dass jede Form der körperlichen Einführung dieser Produkte in Menschen oder Tiere gesetzlich strikt untersagt ist. Es ist unerlässlich, sich an diese Richtlinien zu halten, um die Einhaltung rechtlicher und ethischer Standards in Forschung und Experiment zu gewährleisten.