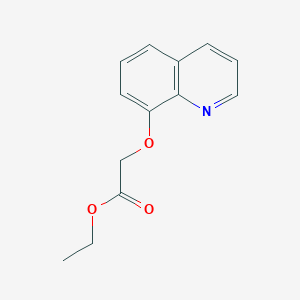
Ethyl (quinolin-8-yloxy)acetate
Übersicht
Beschreibung
Ethyl (quinolin-8-yloxy)acetate is an organic compound that belongs to the class of quinoline derivatives Quinoline derivatives are known for their wide range of biological activities and are used in various fields such as medicine, agriculture, and materials science
Vorbereitungsmethoden
Synthetic Routes and Reaction Conditions: Ethyl (quinolin-8-yloxy)acetate can be synthesized through several methods, including conventional heating, microwave irradiation, and ultrasound irradiation. One common method involves the reaction of 8-hydroxyquinoline with ethyl chloroacetate in the presence of a base such as potassium carbonate. The reaction is typically carried out in a solvent like acetone under reflux conditions .
Industrial Production Methods: In industrial settings, the synthesis of this compound may be optimized for higher yields and efficiency. Microwave-assisted synthesis has gained popularity due to its ability to accelerate reaction rates and improve product yields. This method involves the use of microwave irradiation to heat the reaction mixture, leading to faster and more efficient synthesis compared to conventional methods .
Analyse Chemischer Reaktionen
Types of Reactions: Ethyl (quinolin-8-yloxy)acetate undergoes various chemical reactions, including substitution, oxidation, and reduction. One notable reaction is the Williamson ether synthesis, where it can react with halogenated compounds to form bisquinoline derivatives .
Common Reagents and Conditions: Common reagents used in the reactions of this compound include bases like potassium carbonate and solvents such as acetone and acetonitrile. Reaction conditions often involve refluxing the mixture to ensure complete reaction .
Major Products Formed: The major products formed from the reactions of this compound depend on the specific reaction conditions and reagents used. For example, in the Williamson ether synthesis, bisquinoline derivatives are formed .
Wissenschaftliche Forschungsanwendungen
Ethyl (quinolin-8-yloxy)acetate has several scientific research applications, particularly in the fields of chemistry, biology, and medicine. In chemistry, it is used as a building block for the synthesis of more complex molecules. In biology and medicine, it has been studied for its potential anticancer and antimicrobial properties. Researchers have explored its use as a tubulin polymerization inhibitor, which can interfere with cell division and potentially lead to the development of new anticancer drugs .
Wirkmechanismus
The mechanism of action of ethyl (quinolin-8-yloxy)acetate involves its interaction with molecular targets such as tubulin. By inhibiting tubulin polymerization, it disrupts the formation of microtubules, which are essential for cell division. This disruption can lead to cell cycle arrest and apoptosis (programmed cell death) in cancer cells .
Vergleich Mit ähnlichen Verbindungen
Ethyl (quinolin-8-yloxy)acetate can be compared to other quinoline derivatives such as (quinolin-8-yloxy)-acetic acid, (2-methyl-quinolin-4-yloxy)-acetic acid, and 2-(quinolin-3-yl)acetic acid. These compounds share similar structural features but may differ in their biological activities and applications.
Conclusion
This compound is a versatile compound with significant potential in various scientific fields. Its synthesis can be achieved through multiple methods, and it undergoes a range of chemical reactions. Its applications in research, particularly in the development of new anticancer agents, highlight its importance in medicinal chemistry. By understanding its mechanism of action and comparing it with similar compounds, researchers can further explore its potential and develop new applications.
Eigenschaften
IUPAC Name |
ethyl 2-quinolin-8-yloxyacetate | |
---|---|---|
Details | Computed by LexiChem 2.6.6 (PubChem release 2019.06.18) | |
Source | PubChem | |
URL | https://pubchem.ncbi.nlm.nih.gov | |
Description | Data deposited in or computed by PubChem | |
InChI |
InChI=1S/C13H13NO3/c1-2-16-12(15)9-17-11-7-3-5-10-6-4-8-14-13(10)11/h3-8H,2,9H2,1H3 | |
Details | Computed by InChI 1.0.5 (PubChem release 2019.06.18) | |
Source | PubChem | |
URL | https://pubchem.ncbi.nlm.nih.gov | |
Description | Data deposited in or computed by PubChem | |
InChI Key |
UMSJLXSVLSTJAP-UHFFFAOYSA-N | |
Details | Computed by InChI 1.0.5 (PubChem release 2019.06.18) | |
Source | PubChem | |
URL | https://pubchem.ncbi.nlm.nih.gov | |
Description | Data deposited in or computed by PubChem | |
Canonical SMILES |
CCOC(=O)COC1=CC=CC2=C1N=CC=C2 | |
Details | Computed by OEChem 2.1.5 (PubChem release 2019.06.18) | |
Source | PubChem | |
URL | https://pubchem.ncbi.nlm.nih.gov | |
Description | Data deposited in or computed by PubChem | |
Molecular Formula |
C13H13NO3 | |
Details | Computed by PubChem 2.1 (PubChem release 2019.06.18) | |
Source | PubChem | |
URL | https://pubchem.ncbi.nlm.nih.gov | |
Description | Data deposited in or computed by PubChem | |
Molecular Weight |
231.25 g/mol | |
Details | Computed by PubChem 2.1 (PubChem release 2021.05.07) | |
Source | PubChem | |
URL | https://pubchem.ncbi.nlm.nih.gov | |
Description | Data deposited in or computed by PubChem | |
Synthesis routes and methods
Procedure details
Haftungsausschluss und Informationen zu In-Vitro-Forschungsprodukten
Bitte beachten Sie, dass alle Artikel und Produktinformationen, die auf BenchChem präsentiert werden, ausschließlich zu Informationszwecken bestimmt sind. Die auf BenchChem zum Kauf angebotenen Produkte sind speziell für In-vitro-Studien konzipiert, die außerhalb lebender Organismen durchgeführt werden. In-vitro-Studien, abgeleitet von dem lateinischen Begriff "in Glas", beinhalten Experimente, die in kontrollierten Laborumgebungen unter Verwendung von Zellen oder Geweben durchgeführt werden. Es ist wichtig zu beachten, dass diese Produkte nicht als Arzneimittel oder Medikamente eingestuft sind und keine Zulassung der FDA für die Vorbeugung, Behandlung oder Heilung von medizinischen Zuständen, Beschwerden oder Krankheiten erhalten haben. Wir müssen betonen, dass jede Form der körperlichen Einführung dieser Produkte in Menschen oder Tiere gesetzlich strikt untersagt ist. Es ist unerlässlich, sich an diese Richtlinien zu halten, um die Einhaltung rechtlicher und ethischer Standards in Forschung und Experiment zu gewährleisten.