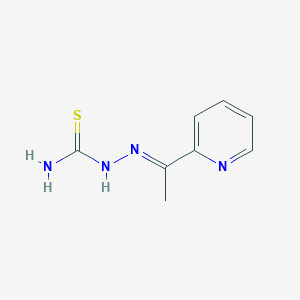
2-Acetylpyridin-thiosemicarbazon
Übersicht
Beschreibung
2-Acetylpyridine thiosemicarbazone is a chemical compound known for its significant biological and pharmacological properties. It belongs to the class of thiosemicarbazones, which are derived from the reaction of thiosemicarbazide with aldehydes or ketones.
Wissenschaftliche Forschungsanwendungen
Anticancer Activity
The anticancer properties of 2-acetylpyridine thiosemicarbazone derivatives have been extensively studied. These compounds exhibit significant cytotoxic effects against various cancer cell lines.
- Mechanisms of Action : Thiosemicarbazones can induce apoptosis in cancer cells through the generation of reactive oxygen species (ROS) and by interfering with cellular signaling pathways. For example, copper(II) complexes of thiosemicarbazones have shown enhanced anti-proliferative activity against lung cancer cells, promoting apoptosis through ROS generation .
- Case Studies : Research indicates that certain derivatives demonstrate selective activity against human lung cancer xenografts without causing cardiotoxicity, making them promising candidates for further development . Additionally, studies have shown that 2-acetylpyridine thiosemicarbazone derivatives can inhibit the proliferation of hepatoblastoma cell lines, showcasing their potential as effective anti-tumor agents .
Antimalarial Properties
2-Acetylpyridine thiosemicarbazones have also been investigated for their antimalarial properties.
- Efficacy : Compounds like 2-acetylpyridine 4-phenyl-3-thiosemicarbazone have been found to exhibit significant activity against Plasmodium berghei in murine models. The presence of specific substituents on the thiosemicarbazone moiety was critical for enhancing antimalarial activity .
- Research Findings : In vivo studies demonstrated that certain derivatives could produce cures at low dosages (as low as 20 mg/kg), indicating their potential for therapeutic use in malaria treatment .
Antiviral Activity
The antiviral potential of 2-acetylpyridine thiosemicarbazones has been explored, particularly against herpes simplex virus types 1 and 2.
- Inhibition Mechanism : These compounds have been shown to inhibit viral replication more effectively than some cellular controls, suggesting a direct antiviral mechanism . The structural features of thiosemicarbazones contribute to their ability to interfere with viral life cycles.
Antibacterial Activity
The antibacterial properties of 2-acetylpyridine thiosemicarbazones are noteworthy, particularly against Gram-positive bacteria.
- Activity Spectrum : Studies indicate that while these compounds are ineffective against Gram-negative bacteria, they exhibit high activity against strains such as Staphylococcus aureus, with minimal inhibitory concentrations (MICs) as low as 1 µg/ml . This highlights their potential as lead compounds for developing new antibacterial agents.
Metal Complexes and Enhanced Activity
The formation of metal complexes with thiosemicarbazones significantly enhances their biological activities.
- Copper(II) Complexes : These complexes have shown strong anticancer activity and can also enhance the stability and solubility of the parent compounds. For instance, copper(II) complexes have been linked to increased cytotoxicity against various cancer cell lines compared to their free ligand counterparts .
Wirkmechanismus
Target of Action
Thiosemicarbazones, including 2-Acetylpyridine thiosemicarbazone, are known to have substantial biological relevance . They often act as ligands to generate highly stable metal–organic complexes . The primary targets of these compounds are transition metals present in biological systems . These compounds can combine with the same metal ions in vivo, producing synergetic results and enhancing the potency of the formed complex .
Mode of Action
The mode of action of 2-Acetylpyridine thiosemicarbazone involves its interaction with its targets, primarily metal ions. The gold (III) complexes probably act by inhibiting the activity of thioredoxin reductase enzyme whereas the mode of action of the platinum (II,IV) complexes involves binding to DNA . The coordination chemistry of thiosemicarbazone complexes can vary upon changing aldehydes and ketones, substituents attached to the carbonyl moiety, metal and its oxidation state, geometries, counter ions, presence of a solvent or additional molecules in the structures and substituents on the S or N (4)-atoms .
Biochemical Pathways
The biochemical pathways affected by 2-Acetylpyridine thiosemicarbazone are primarily related to its interaction with metal ions. The coordination of thiosemicarbazones to metal ions results in improved pharmacological activities
Pharmacokinetics
The pharmacokinetic properties of thiosemicarbazones and their metal complexes, in general, are known to be influenced by factors such as the type of carbonyl compound (aldehydes or ketones), substituents attached to the carbonyl moiety, the metal ion and its oxidation state, geometries, counter ions, presence of a solvent or additional molecules in the structures and substituents on the s or n (4)-atoms .
Result of Action
The result of the action of 2-Acetylpyridine thiosemicarbazone is primarily observed in its cytotoxic activities. For instance, certain complexes of 2-Acetylpyridine thiosemicarbazone have shown significant antitumor activity against human malignant glioma cell lines . Cells treated with these compounds presented morphological changes such as cell shrinkage and blebs formation, which indicate cell death by apoptosis induction .
Action Environment
The action environment of 2-Acetylpyridine thiosemicarbazone is primarily within biological systems where metal ions are present. The coordination chemistry of thiosemicarbazone complexes can vary in different coordination environments . Environmental factors such as pH, the presence of oxidant reactants or the establishment of redox processes, the effects of the solvent, the temperature, and the electromagnetic radiation can influence the desulfurization reactions of thiosemicarbazones .
Biochemische Analyse
Biochemical Properties
2-Acetylpyridine Thiosemicarbazone interacts with various enzymes, proteins, and other biomolecules. It has been found that thiosemicarbazones can act as strong RR inhibitors . The ability of the transition metals to acquire different geometries in different coordination environments has encouraged researchers to explore the coordination chemistry of thiosemicarbazone complexes .
Cellular Effects
2-Acetylpyridine Thiosemicarbazone has shown significant cytotoxic activity against malignant glioblastoma and breast cancer cells at nanomolar concentrations . It induces morphological changes characteristic of apoptotic death in tumor cells and shows no toxicity against erythrocytes .
Molecular Mechanism
The molecular mechanism of action of 2-Acetylpyridine Thiosemicarbazone involves its complexation with metal ions. For instance, copper(II) complexes with isomeric morpholine-thiosemicarbazone hybrid ligands show good cytotoxicity in cancer cells, and the molecular target responsible for this activity might be tubulin .
Temporal Effects in Laboratory Settings
When 2-Acetylpyridine Thiosemicarbazone was added to nickel (II) in a sodium acetate-acetic acid pH 6 solution, a yellow-greenish solution was generated almost instantly . This indicates that the compound has a rapid reaction time in laboratory settings.
Dosage Effects in Animal Models
In animal models, the effects of 2-Acetylpyridine Thiosemicarbazone vary with different dosages. In an acute nonclinical toxicological test, animals treated with a certain thiosemicarbazone showed behavioral changes of stimulus of the central nervous system at 300 mg/kg .
Metabolic Pathways
The metabolic pathways of 2-Acetylpyridine Thiosemicarbazone involve dehydrogenation, hydroxylation, oxidative desulfuration (to semicarbazone and amidrazone), and demethylation . These reactions can vary depending on the presence of different metal ions .
Transport and Distribution
It has been suggested that the compound may be transported and distributed via its complexation with metal ions .
Subcellular Localization
It has been suggested that the compound and its complexes may be sequestered within the lysosomes .
Vorbereitungsmethoden
Synthetic Routes and Reaction Conditions: The synthesis of 2-acetylpyridine thiosemicarbazone typically involves the condensation of 2-acetylpyridine with thiosemicarbazide. The reaction is usually carried out in an ethanol solution under reflux conditions. The product is then purified through recrystallization .
Industrial Production Methods: While specific industrial production methods for 2-acetylpyridine thiosemicarbazone are not extensively documented, the general approach involves large-scale synthesis using similar reaction conditions as in laboratory settings. The process may be optimized for higher yields and purity through controlled reaction parameters and advanced purification techniques .
Analyse Chemischer Reaktionen
Types of Reactions: 2-Acetylpyridine thiosemicarbazone undergoes various chemical reactions, including:
Oxidation: It can be oxidized to form corresponding sulfoxides or sulfones.
Reduction: Reduction reactions can convert it into thiosemicarbazides.
Substitution: It can participate in nucleophilic substitution reactions, particularly at the nitrogen or sulfur atoms.
Common Reagents and Conditions:
Oxidation: Common oxidizing agents include hydrogen peroxide and potassium permanganate.
Reduction: Reducing agents such as sodium borohydride or lithium aluminum hydride are often used.
Substitution: Reagents like alkyl halides or acyl chlorides can be employed under basic or acidic conditions.
Major Products Formed:
Oxidation: Sulfoxides and sulfones.
Reduction: Thiosemicarbazides.
Substitution: Various substituted thiosemicarbazones.
Vergleich Mit ähnlichen Verbindungen
- 2-Benzoylpyridine N(4)-methyl thiosemicarbazone
- 2-Acetylpyridine N(4)-methylthiosemicarbazone
- 2-Hydroxyacetophenone thiosemicarbazone
Comparison: 2-Acetylpyridine thiosemicarbazone is unique due to its specific structural features that enhance its binding affinity with metals and its biological activity. Compared to similar compounds, it has shown higher efficacy in antiviral and anticancer applications. The presence of the pyridine ring in its structure contributes to its stability and reactivity, making it a versatile compound in various research fields .
Biologische Aktivität
2-Acetylpyridine thiosemicarbazone (2-APT) is a compound of significant interest in medicinal chemistry, particularly for its anti-cancer properties. This article explores its biological activities, mechanisms of action, and potential therapeutic applications, supported by various studies and data.
Chemical Structure and Properties
2-Acetylpyridine thiosemicarbazone is derived from the condensation of 2-acetylpyridine with thiosemicarbazide. The resulting compound features a thiosemicarbazone functional group, which is known for its ability to form coordination complexes with metal ions. This property is crucial for its biological activity, particularly in cancer therapy.
The biological activity of 2-APT primarily stems from several mechanisms:
- Inhibition of Ribonucleotide Reductase: 2-APT has been shown to inhibit ribonucleotide reductase, an enzyme critical for DNA synthesis, thereby exerting anti-cancer effects by hindering cell proliferation .
- Induction of Apoptosis: The compound induces apoptosis in cancer cells through the generation of reactive oxygen species (ROS) and disruption of lysosomal integrity. This leads to lysosomal membrane permeabilization (LMP), which is a precursor to apoptotic cell death .
- Metal Chelation: 2-APT acts as a metal chelator, forming complexes with transition metals like copper(II) and nickel(II). These complexes enhance the cytotoxicity against various cancer cell lines by promoting ROS generation and apoptosis .
Anticancer Activity
Numerous studies have demonstrated the anticancer potential of 2-APT:
- Cell Line Studies: 2-APT exhibits significant cytotoxicity against various cancer cell lines, including lung (A549), prostate (PC3), and glioma cells. For instance, a study reported an IC50 value of approximately 0.20 mM against A549 cells .
- In Vivo Studies: In animal models, 2-APT has shown promising results in reducing tumor size without causing significant cardiotoxicity, making it a candidate for further development as an anti-cancer agent .
Comparative Studies
A comparative analysis of 2-APT with other thiosemicarbazones reveals its unique efficacy:
Case Study 1: Antitumor Efficacy in Lung Cancer
A study conducted by Lovejoy et al. highlighted the effectiveness of 2-APT in inhibiting lung cancer cell growth both in vitro and in vivo. The compound demonstrated a selective action on cancer cells while sparing normal cells, indicating a favorable therapeutic index .
Case Study 2: Mechanistic Insights into Apoptosis Induction
Research by Zhao et al. provided insights into the mechanism by which 2-APT induces apoptosis through ROS generation. Their findings suggest that the compound's ability to disrupt lysosomal function plays a crucial role in triggering apoptotic pathways .
Eigenschaften
IUPAC Name |
[(E)-1-pyridin-2-ylethylideneamino]thiourea | |
---|---|---|
Source | PubChem | |
URL | https://pubchem.ncbi.nlm.nih.gov | |
Description | Data deposited in or computed by PubChem | |
InChI |
InChI=1S/C8H10N4S/c1-6(11-12-8(9)13)7-4-2-3-5-10-7/h2-5H,1H3,(H3,9,12,13)/b11-6+ | |
Source | PubChem | |
URL | https://pubchem.ncbi.nlm.nih.gov | |
Description | Data deposited in or computed by PubChem | |
InChI Key |
RIFVPOHZBSIFRL-IZZDOVSWSA-N | |
Source | PubChem | |
URL | https://pubchem.ncbi.nlm.nih.gov | |
Description | Data deposited in or computed by PubChem | |
Canonical SMILES |
CC(=NNC(=S)N)C1=CC=CC=N1 | |
Source | PubChem | |
URL | https://pubchem.ncbi.nlm.nih.gov | |
Description | Data deposited in or computed by PubChem | |
Isomeric SMILES |
C/C(=N\NC(=S)N)/C1=CC=CC=N1 | |
Source | PubChem | |
URL | https://pubchem.ncbi.nlm.nih.gov | |
Description | Data deposited in or computed by PubChem | |
Molecular Formula |
C8H10N4S | |
Source | PubChem | |
URL | https://pubchem.ncbi.nlm.nih.gov | |
Description | Data deposited in or computed by PubChem | |
DSSTOX Substance ID |
DTXSID101197659 | |
Record name | (2E)-2-[1-(2-Pyridinyl)ethylidene]hydrazinecarbothioamide | |
Source | EPA DSSTox | |
URL | https://comptox.epa.gov/dashboard/DTXSID101197659 | |
Description | DSSTox provides a high quality public chemistry resource for supporting improved predictive toxicology. | |
Molecular Weight |
194.26 g/mol | |
Source | PubChem | |
URL | https://pubchem.ncbi.nlm.nih.gov | |
Description | Data deposited in or computed by PubChem | |
CAS No. |
142564-62-3 | |
Record name | (2E)-2-[1-(2-Pyridinyl)ethylidene]hydrazinecarbothioamide | |
Source | CAS Common Chemistry | |
URL | https://commonchemistry.cas.org/detail?cas_rn=142564-62-3 | |
Description | CAS Common Chemistry is an open community resource for accessing chemical information. Nearly 500,000 chemical substances from CAS REGISTRY cover areas of community interest, including common and frequently regulated chemicals, and those relevant to high school and undergraduate chemistry classes. This chemical information, curated by our expert scientists, is provided in alignment with our mission as a division of the American Chemical Society. | |
Explanation | The data from CAS Common Chemistry is provided under a CC-BY-NC 4.0 license, unless otherwise stated. | |
Record name | Hydrazinecarbothioamide, 2-(1-(2-pyridinyl)ethylidene)-, (E)- | |
Source | ChemIDplus | |
URL | https://pubchem.ncbi.nlm.nih.gov/substance/?source=chemidplus&sourceid=0142564623 | |
Description | ChemIDplus is a free, web search system that provides access to the structure and nomenclature authority files used for the identification of chemical substances cited in National Library of Medicine (NLM) databases, including the TOXNET system. | |
Record name | (2E)-2-[1-(2-Pyridinyl)ethylidene]hydrazinecarbothioamide | |
Source | EPA DSSTox | |
URL | https://comptox.epa.gov/dashboard/DTXSID101197659 | |
Description | DSSTox provides a high quality public chemistry resource for supporting improved predictive toxicology. | |
Retrosynthesis Analysis
AI-Powered Synthesis Planning: Our tool employs the Template_relevance Pistachio, Template_relevance Bkms_metabolic, Template_relevance Pistachio_ringbreaker, Template_relevance Reaxys, Template_relevance Reaxys_biocatalysis model, leveraging a vast database of chemical reactions to predict feasible synthetic routes.
One-Step Synthesis Focus: Specifically designed for one-step synthesis, it provides concise and direct routes for your target compounds, streamlining the synthesis process.
Accurate Predictions: Utilizing the extensive PISTACHIO, BKMS_METABOLIC, PISTACHIO_RINGBREAKER, REAXYS, REAXYS_BIOCATALYSIS database, our tool offers high-accuracy predictions, reflecting the latest in chemical research and data.
Strategy Settings
Precursor scoring | Relevance Heuristic |
---|---|
Min. plausibility | 0.01 |
Model | Template_relevance |
Template Set | Pistachio/Bkms_metabolic/Pistachio_ringbreaker/Reaxys/Reaxys_biocatalysis |
Top-N result to add to graph | 6 |
Feasible Synthetic Routes
Q1: What is the primary mechanism of action for 2-acetylpyridine thiosemicarbazone and its derivatives?
A1: 2-acetylpyridine thiosemicarbazones primarily exert their effects by chelating iron. [] This iron chelation disrupts critical cellular processes, such as inhibiting ribonucleotide reductase, an enzyme essential for DNA synthesis. [, ] Additionally, some derivatives can induce lysosomal membrane permeabilization, contributing to cytotoxicity. []
Q2: How does iron chelation by 2-acetylpyridine thiosemicarbazones affect tumor cells?
A2: Iron chelation by these compounds leads to decreased intracellular iron levels, which are essential for tumor cell growth and proliferation. [] This can trigger cell cycle arrest and ultimately lead to cell death. []
Q3: Do 2-acetylpyridine thiosemicarbazones exhibit selectivity towards cancerous cells?
A3: Some 2-acetylpyridine thiosemicarbazones have shown greater cytotoxicity against resistant cancer cells compared to non-resistant counterparts. [] This selectivity is linked to their interaction with P-glycoprotein (Pgp), a protein overexpressed in many drug-resistant cancers. []
Q4: What is the molecular formula and weight of 2-acetylpyridine thiosemicarbazone?
A4: The molecular formula of 2-acetylpyridine thiosemicarbazone is C8H10N4S, and its molecular weight is 194.26 g/mol.
Q5: Which spectroscopic techniques are commonly employed to characterize 2-acetylpyridine thiosemicarbazones?
A5: Researchers frequently utilize infrared (IR) spectroscopy, ultraviolet-visible (UV-Vis) spectroscopy, nuclear magnetic resonance (NMR) spectroscopy, and mass spectrometry (MS) to characterize these compounds and their metal complexes. [, , , , , , ]
Q6: How do structural modifications at the N4 position of 2-acetylpyridine thiosemicarbazones impact their activity?
A6: Studies have shown that the size and nature of substituents at the N4 position significantly influence activity. [, , , ] For instance, incorporating the N4 nitrogen into a six or seven-membered ring system, like piperidine or azabicyclo[3.2.2]nonane, can enhance antimalarial potency. []
Q7: What is the role of the substituent on the azomethine carbon in determining the activity of 2-acetylpyridine thiosemicarbazones?
A7: The size of the aliphatic substituent on the azomethine carbon plays a role in antimalarial activity, with larger substituents generally leading to reduced potency. []
Q8: What is the stability profile of 2-acetylpyridine thiosemicarbazone and its complexes?
A8: The stability of these compounds can vary depending on the specific derivative and the presence of metal ions. Some complexes, like the 191Os-labeled 2-acetylpyridine 4-N-methylthiosemicarbazone (191Os-APMTS), exhibit high stability in formulation and human serum for extended periods. []
Q9: Are there specific formulation strategies employed to enhance the stability or bioavailability of 2-acetylpyridine thiosemicarbazones?
A9: Researchers are exploring various strategies to optimize the delivery and efficacy of these compounds. For instance, complexation with zinc(II) has been shown to enhance cytotoxicity and enable lysosomal targeting. []
Q10: Against which pathogens have 2-acetylpyridine thiosemicarbazones demonstrated in vitro activity?
A10: These compounds have shown promising in vitro activity against various pathogens, including Trypanosoma rhodesiense, Plasmodium falciparum, Neisseria gonorrhoeae, Mycobacterium tuberculosis, and Candida albicans. [, , , , , , ]
Q11: Have any 2-acetylpyridine thiosemicarbazones progressed to clinical trials?
A11: Yes, the zinc(II) complex of di-2-pyridylketone 4-cyclohexyl-4-methyl-3-thiosemicarbazone (DpC), a compound structurally related to 2-acetylpyridine thiosemicarbazone, is scheduled to enter clinical trials for its anticancer properties. []
Q12: Are there known resistance mechanisms against 2-acetylpyridine thiosemicarbazones?
A12: While resistance mechanisms can develop, some derivatives, like those with electron-withdrawing groups on the imine carbon, can overcome Pgp-mediated drug resistance in cancer cells. []
Haftungsausschluss und Informationen zu In-Vitro-Forschungsprodukten
Bitte beachten Sie, dass alle Artikel und Produktinformationen, die auf BenchChem präsentiert werden, ausschließlich zu Informationszwecken bestimmt sind. Die auf BenchChem zum Kauf angebotenen Produkte sind speziell für In-vitro-Studien konzipiert, die außerhalb lebender Organismen durchgeführt werden. In-vitro-Studien, abgeleitet von dem lateinischen Begriff "in Glas", beinhalten Experimente, die in kontrollierten Laborumgebungen unter Verwendung von Zellen oder Geweben durchgeführt werden. Es ist wichtig zu beachten, dass diese Produkte nicht als Arzneimittel oder Medikamente eingestuft sind und keine Zulassung der FDA für die Vorbeugung, Behandlung oder Heilung von medizinischen Zuständen, Beschwerden oder Krankheiten erhalten haben. Wir müssen betonen, dass jede Form der körperlichen Einführung dieser Produkte in Menschen oder Tiere gesetzlich strikt untersagt ist. Es ist unerlässlich, sich an diese Richtlinien zu halten, um die Einhaltung rechtlicher und ethischer Standards in Forschung und Experiment zu gewährleisten.