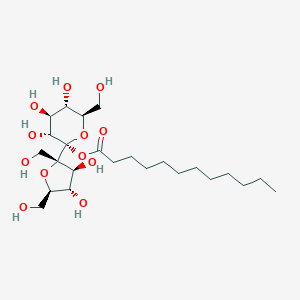
Saccharoselaurat
- Klicken Sie auf QUICK INQUIRY, um ein Angebot von unserem Expertenteam zu erhalten.
- Mit qualitativ hochwertigen Produkten zu einem WETTBEWERBSFÄHIGEN Preis können Sie sich mehr auf Ihre Forschung konzentrieren.
Übersicht
Beschreibung
Sucrose laurate is a type of sucrose ester, which is a non-ionic surfactant. It is formed by the esterification of sucrose with lauric acid. Sucrose laurate is widely used in various industries due to its excellent emulsifying, stabilizing, and surfactant properties. It is commonly found in food, cosmetics, and pharmaceutical products.
Wissenschaftliche Forschungsanwendungen
Sucrose laurate has a wide range of applications in scientific research:
Wirkmechanismus
Target of Action
Sucrose laurate, also known as [(2S,3R,4S,5S,6R)-2-[(2S,3S,4S,5R)-3,4-dihydroxy-2,5-bis(hydroxymethyl)oxolan-2-yl]-3,4,5-trihydroxy-6-(hydroxymethyl)oxan-2-yl] dodecanoate, primarily targets the intestinal epithelial cells . It interacts with the plasma membrane and tight junction proteins of these cells, such as ZO-1 .
Mode of Action
Sucrose laurate acts as a permeation enhancer (PE), working to reversibly enhance epithelial permeability via the paracellular route . It increases the apparent permeability coefficient (Papp) of certain substances and reduces transepithelial electrical resistance (TEER) across monolayers . This compound alters the expression of the tight junction protein, ZO-1, increases plasma membrane potential, and decreases mitochondrial membrane potential in Caco-2 cells .
Biochemical Pathways
Sucrose laurate affects multiple biochemical pathways. It has been found to down-regulate cell wall-associated hydrolase, inhibit the synthesis of fatty acids, influence nucleic acid synthesis, disturb amino acid metabolism, and block the HMP pathway and TCA cycle .
Pharmacokinetics
Sucrose laurate’s ADME properties significantly impact its bioavailability. In rat jejunal and colonic instillations, 50 and 100 mM sucrose laurate co-administered with insulin induced blood glucose reductions and achieved relative bioavailability values of 2.4% and 8.9%, respectively . This is on par with the gold standard PE, sodium caprate (C10) .
Result of Action
The primary result of sucrose laurate’s action is plasma membrane perturbation, leading to tight junction openings and a predominant paracellular flux . This results in increased permeability and enhanced absorption of certain substances, including macromolecules . It also causes the leakage of intracellular materials .
Action Environment
The action of sucrose laurate can be influenced by environmental factors. For instance, the concentration of sucrose laurate plays a crucial role in its efficacy . Higher concentrations can induce cytotoxicity, while lower concentrations can enhance permeability . Furthermore, temperature can affect the production yield and selectivity of sucrose laurate .
Biochemische Analyse
Biochemical Properties
Sucrose laurate plays a key role in biochemical reactions. It acts as an emulsifier, enabling the mixing of water and oil-based components in cosmetics . It also serves as a skin conditioning agent, an emollient, and a cleanser . In the pharmaceutical industry, it aids in the solubilization and absorption of active pharmaceutical ingredients, ensuring their effectiveness . Moreover, it can enhance the bioavailability of poorly soluble drugs, making them more readily absorbed by the body .
Cellular Effects
Sucrose laurate has various effects on different types of cells and cellular processes. For instance, it has been shown to increase the apparent permeability coefficient (Papp) of [14C]-mannitol and reduce transepithelial electrical resistance (TEER) across Caco-2 monolayers . It alters the expression of the tight junction protein, ZO-1, increases plasma membrane potential, and decreases mitochondrial membrane potential in Caco-2 cells .
Molecular Mechanism
The primary action of Sucrose laurate is plasma membrane perturbation, leading to tight junction openings and a predominant paracellular flux . This mechanism allows Sucrose laurate to effectively lift away dirt, makeup, and excess sebum without disrupting the skin’s natural lipid barrier .
Dosage Effects in Animal Models
The effects of Sucrose laurate vary with different dosages in animal models. For instance, in rat jejunal and colonic instillations, 50 and 100 mM Sucrose laurate co-administered with insulin induced blood glucose reductions .
Metabolic Pathways
Sucrose laurate is involved in the esterification reaction where sugar is reacted with free fatty acid under a homogenous acid catalyst to produce sugar ester and water . This process is generally carried out under reduced pressure and anhydrous conditions .
Transport and Distribution
Its ability to act as an emulsifier, enabling the mixing of water and oil-based components, suggests that it may be distributed in both hydrophilic and lipophilic environments .
Subcellular Localization
Given its role as an emulsifier and its impact on cellular processes such as the disruption of tight junctions , it is likely that Sucrose laurate interacts with various cellular compartments.
Vorbereitungsmethoden
Synthetic Routes and Reaction Conditions: Sucrose laurate can be synthesized through a two-stage process. In the first stage, lauric acid is esterified with methanol to produce methyl laurate. This reaction is typically catalyzed by Amberlyst 15, a strong acid ion-exchange resin, at a temperature of 110°C with a residence time of 5 minutes . In the second stage, methyl laurate undergoes transesterification with sucrose to form sucrose laurate. This process is carried out under reduced pressure and anhydrous conditions to achieve high selectivity and yield .
Industrial Production Methods: Industrial production of sucrose laurate often involves the use of ultrasonic frequencies to enhance the reaction efficiency. Ultrasonic waves can reduce reaction temperature and pressure, improve yield, and shorten reaction time . The process involves the transesterification of sucrose with lauric acid or its esters under ultrasonic conditions, resulting in a mixture of sucrose monolaurate, dilaurate, and polylaurate .
Analyse Chemischer Reaktionen
Types of Reactions: Sucrose laurate primarily undergoes esterification and transesterification reactions. It can also participate in hydrolysis and saponification reactions under certain conditions .
Common Reagents and Conditions:
Esterification: Lauric acid and methanol with Amberlyst 15 as a catalyst at 110°C.
Transesterification: Methyl laurate and sucrose under reduced pressure and anhydrous conditions.
Hydrolysis and Saponification: These reactions typically occur in the presence of water and a base, such as sodium hydroxide.
Major Products:
Esterification: Methyl laurate.
Transesterification: Sucrose monolaurate, dilaurate, and polylaurate.
Hydrolysis and Saponification: Lauric acid and sucrose.
Vergleich Mit ähnlichen Verbindungen
Sucrose laurate is compared with other sucrose esters such as sucrose stearate and sucrose palmitate. While all these compounds are used as surfactants and emulsifiers, sucrose laurate is unique in its ability to enhance intestinal permeability and facilitate drug absorption . Similar compounds include:
Sucrose stearate: Used in cosmetics and pharmaceuticals for its emulsifying properties.
Sucrose palmitate: Commonly used in food products as an emulsifier.
Sucrose laurate stands out due to its specific applications in drug delivery and its effectiveness as an intestinal permeation enhancer .
Eigenschaften
CAS-Nummer |
25339-99-5 |
---|---|
Molekularformel |
C24H46O13 |
Molekulargewicht |
542.6 g/mol |
IUPAC-Name |
2-[(2S)-3,4-dihydroxy-2,5-bis(hydroxymethyl)oxolan-2-yl]oxy-6-(hydroxymethyl)oxane-3,4,5-triol;dodecanoic acid |
InChI |
InChI=1S/C12H22O11.C12H24O2/c13-1-4-6(16)8(18)9(19)11(21-4)23-12(3-15)10(20)7(17)5(2-14)22-12;1-2-3-4-5-6-7-8-9-10-11-12(13)14/h4-11,13-20H,1-3H2;2-11H2,1H3,(H,13,14)/t4?,5?,6?,7?,8?,9?,10?,11?,12-;/m0./s1 |
InChI-Schlüssel |
PVVVEHXCVQRLOC-NSIFZURYSA-N |
SMILES |
CCCCCCCCCCCC(=O)OC1(C(C(C(C(O1)CO)O)O)O)C2(C(C(C(O2)CO)O)O)CO |
Isomerische SMILES |
CCCCCCCCCCCC(=O)O.C(C1C(C(C(C(O1)O[C@]2(C(C(C(O2)CO)O)O)CO)O)O)O)O |
Kanonische SMILES |
CCCCCCCCCCCC(=O)O.C(C1C(C(C(C(O1)OC2(C(C(C(O2)CO)O)O)CO)O)O)O)O |
Key on ui other cas no. |
25339-99-5 |
Piktogramme |
Corrosive |
Verwandte CAS-Nummern |
37266-93-6 (unspecified laurate) |
Synonyme |
eta-fructofuranosyl-alpha-D-glucopyranoside monododecanoate L-1690 sucrose laurate sucrose monododecanoate sucrose monolaurate |
Herkunft des Produkts |
United States |
Haftungsausschluss und Informationen zu In-Vitro-Forschungsprodukten
Bitte beachten Sie, dass alle Artikel und Produktinformationen, die auf BenchChem präsentiert werden, ausschließlich zu Informationszwecken bestimmt sind. Die auf BenchChem zum Kauf angebotenen Produkte sind speziell für In-vitro-Studien konzipiert, die außerhalb lebender Organismen durchgeführt werden. In-vitro-Studien, abgeleitet von dem lateinischen Begriff "in Glas", beinhalten Experimente, die in kontrollierten Laborumgebungen unter Verwendung von Zellen oder Geweben durchgeführt werden. Es ist wichtig zu beachten, dass diese Produkte nicht als Arzneimittel oder Medikamente eingestuft sind und keine Zulassung der FDA für die Vorbeugung, Behandlung oder Heilung von medizinischen Zuständen, Beschwerden oder Krankheiten erhalten haben. Wir müssen betonen, dass jede Form der körperlichen Einführung dieser Produkte in Menschen oder Tiere gesetzlich strikt untersagt ist. Es ist unerlässlich, sich an diese Richtlinien zu halten, um die Einhaltung rechtlicher und ethischer Standards in Forschung und Experiment zu gewährleisten.