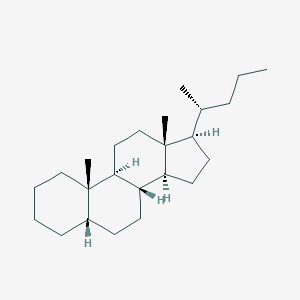
5beta-Cholane
Übersicht
Beschreibung
5beta-Cholane is a triterpene compound that exists as one of the stereoisomers of cholane. Its name is derived from the Greek word “chole,” meaning bile, as it was originally discovered in the bile of the American bullfrog (Rana catesbeiana)
Vorbereitungsmethoden
The preparation of 5beta-Cholane and its derivatives involves several synthetic routes. One method includes the oxidation of chenodeoxycholic acid using hydrogen peroxide in the presence of an acid catalyst to obtain 3alpha-hydroxy-7-oxo-5beta-cholanic acid . This method is advantageous due to its mild reaction conditions, high yield, and low pollution.
Industrial production methods for this compound typically involve the extraction and purification from natural sources, followed by chemical modifications to obtain the desired derivatives .
Analyse Chemischer Reaktionen
5beta-Cholane undergoes various chemical reactions, including:
Oxidation: For example, the oxidation of chenodeoxycholic acid to produce 3alpha-hydroxy-7-oxo-5beta-cholanic acid.
Reduction: Reduction reactions can modify the functional groups on the cholane backbone.
Common reagents used in these reactions include hydrogen peroxide for oxidation and tosyl chloride for substitution reactions. The major products formed from these reactions are various functionalized derivatives of this compound.
Wissenschaftliche Forschungsanwendungen
Scientific Research Applications
5beta-Cholane and its derivatives have several key applications:
1. Cholesterol Regulation
Research indicates that this compound has hypocholesterolemic effects, which means it can help lower cholesterol levels in the body. This effect is primarily due to its ability to modulate bile acid transport and metabolism, influencing lipid absorption and excretion.
2. Bile Acid Research
As a precursor in the synthesis of bile acids, this compound is essential for the digestion and absorption of dietary fats. It serves as a reference standard in studies exploring bile acid metabolism.
3. Farnesoid X Receptor Activation
this compound is investigated for its potential as an activator of the farnesoid X receptor (FXR), a nuclear receptor involved in regulating bile acid synthesis, lipid metabolism, and glucose homeostasis. FXR activation has implications for treating metabolic disorders, liver diseases, and cardiovascular conditions .
4. Drug Delivery Systems
In industrial applications, derivatives of this compound are utilized in the development of nanoparticles for targeted drug delivery and cancer imaging. These nanoparticles can enhance the efficacy of therapeutic agents while minimizing side effects .
Case Study 1: FXR Activation
A study conducted by researchers at the University of Tokyo evaluated the FXR agonistic activity of various bile alcohols, including this compound derivatives. The results indicated that certain hydroxylated forms of this compound exhibited significant FXR activation, leading to improved lipid profiles in animal models .
Case Study 2: Cholesterol Management
In clinical trials focusing on non-alcoholic fatty liver disease (NAFLD), patients treated with formulations containing this compound derivatives showed marked improvements in cholesterol levels and liver function tests. This suggests potential therapeutic benefits for managing cholesterol-related disorders.
Table 1: Comparison of Biological Activities
Compound | FXR Activation | Hypocholesterolemic Effect | Application Area |
---|---|---|---|
This compound | Moderate | Yes | Cholesterol Regulation |
This compound-3alpha,7beta | High | Yes | Bile Acid Research |
NMGCA (Derivative) | Very High | Moderate | Metabolic Disorders |
Table 2: Synthesis Methods
Method | Description | Yield (%) |
---|---|---|
Hydroxylation | Conversion of bile acids using ethyl chloroformate | 75-85 |
Chemical Modification | Structural modification to enhance biological activity | Variable |
Wirkmechanismus
The mechanism of action of 5beta-Cholane involves its interaction with various molecular targets and pathways. For instance, it acts as an activator of the farnesol X receptor, influencing gene expression related to cholesterol and lipid metabolism . Additionally, its derivatives can interact with cellular receptors and enzymes, affecting biological activities such as bile acid metabolism .
Vergleich Mit ähnlichen Verbindungen
5beta-Cholane is unique due to its specific stereochemistry and biological activity. Similar compounds include:
5alpha-Cholane: Another stereoisomer of cholane with different biological properties.
24-Nor-5beta-cholane-3alpha,7alpha,12alpha,23-tetrol: A bile acid derivative with distinct structural features.
This compound-3alpha,7alpha,12alpha,24-tetrol: Another bile acid derivative with unique functional groups.
These compounds share a similar cholane backbone but differ in their functional groups and stereochemistry, leading to varied biological activities and applications.
Biologische Aktivität
5β-Cholane, a bile alcohol derived from cholesterol, has garnered significant attention due to its biological activities, particularly its role as a ligand for various receptors involved in metabolic regulation. This article explores the compound's biological activity, focusing on its mechanisms of action, structure-activity relationships, and potential therapeutic applications.
Overview of 5β-Cholane
5β-Cholane is a tetracyclic compound that serves as a precursor to bile acids. It possesses a steroidal structure characterized by four fused rings, which is essential for its interaction with biological receptors. The compound's variations, including hydroxylation at specific positions, significantly influence its biological properties.
5β-Cholane primarily exerts its biological effects through activation of G protein-coupled receptors (GPCRs), notably TGR5 (also known as GPBAR1). Activation of TGR5 leads to increased intracellular cAMP levels, which are crucial for various signaling pathways associated with metabolism and energy homeostasis.
TGR5 Activation
- Receptor Activation : Studies have shown that 5β-cholane derivatives with hydroxyl groups at specific positions (e.g., C-22 to C-26) exhibit enhanced TGR5 agonist activity. For instance, 5β-cholestane-3α,7α,12α,26-tetrol demonstrated comparable luciferase activity to lithocholic acid (LCA), a potent TGR5 agonist .
- cAMP Production : The ability of 5β-cholane derivatives to stimulate cAMP production correlates with their TGR5 activity. Increased hydroxylation in the side chain enhances this effect, suggesting a structure-activity relationship where the number and position of hydroxyl groups are critical for receptor interaction .
Structure-Activity Relationships
The biological activity of 5β-cholane derivatives is significantly influenced by their structural modifications. Research indicates that:
- Hydroxyl Group Positioning : The position of hydroxyl groups on the steroid backbone alters the affinity and efficacy for TGR5. For example, the presence of hydroxyl groups at C-22 and C-23 markedly increases agonistic activity compared to their absence .
- Isomer Variability : Different stereoisomers of 5β-cholane derivatives exhibit varying degrees of biological activity. This variability underscores the importance of stereochemistry in drug design and efficacy .
Therapeutic Applications
Given its role in metabolic regulation, 5β-cholane and its derivatives have potential therapeutic applications in several conditions:
- Metabolic Disorders : By activating TGR5, these compounds may help regulate glucose metabolism and energy expenditure, making them candidates for treating obesity and type 2 diabetes .
- Cholesterol Management : Bile acids are known to modulate cholesterol homeostasis. 5β-Cholane derivatives could be explored for their ability to lower cholesterol levels by enhancing bile acid synthesis and secretion .
Case Studies
Several studies have highlighted the potential of 5β-cholane derivatives in clinical settings:
- Cholic Acid Feeding Studies : Historical studies demonstrated that cholic acid effectively suppressed bile acid and cholesterol biosynthesis in patients with hypercholesterolemia. This suggests that related compounds like 5β-cholane could have similar effects on lipid profiles .
- FXR Agonism : Compounds derived from 5β-cholane have been investigated for their ability to activate farnesoid X receptor (FXR), which plays a critical role in bile acid homeostasis and lipid metabolism. Some derivatives have shown promise in preclinical models for managing liver diseases .
Data Summary
The following table summarizes key findings related to the biological activity of 5β-cholane:
Compound | TGR5 Activity | cAMP Production | Potential Applications |
---|---|---|---|
5β-Cholestane-3α,7α,12α-tetrol | High | Significant | Metabolic disorders |
5β-Cholestane-3α,7α,12α,23-tetrol | Moderate | Moderate | Cholesterol management |
Lithocholic Acid | Very High | Very High | Metabolic regulation |
Q & A
Basic Research Questions
Q. What are the established methods for synthesizing and characterizing 5beta-Cholane in laboratory settings?
To synthesize this compound, researchers typically employ steroid backbone modifications via catalytic hydrogenation or enzymatic reduction of precursor compounds like cholestanes. Characterization requires a combination of nuclear magnetic resonance (NMR) spectroscopy (¹H and ¹³C) to confirm stereochemistry, high-resolution mass spectrometry (HRMS) for molecular weight validation, and chromatographic techniques (HPLC or GC) to assess purity (>95%). Experimental protocols must detail reaction conditions (temperature, solvent, catalyst), purification steps, and spectral data interpretation to ensure reproducibility .
Q. How can researchers validate the purity and stability of this compound in experimental systems?
Purity validation involves using differential scanning calorimetry (DSC) to detect melting point consistency (e.g., 76–78°C, as seen in related cholanes) and thermogravimetric analysis (TGA) to assess thermal decomposition . Stability testing under varying pH, temperature, and light exposure conditions should follow ICH guidelines, with degradation products monitored via LC-MS. Documentation must include controlled storage conditions (e.g., inert atmosphere, −20°C) and batch-specific data .
Q. What spectroscopic and chromatographic techniques are most effective for quantifying this compound in biological matrices?
Liquid chromatography-tandem mass spectrometry (LC-MS/MS) with electrospray ionization (ESI) is preferred for high sensitivity in complex matrices. Calibration curves using deuterated internal standards (e.g., d₆-5beta-Cholane) minimize matrix effects. For non-polar environments, gas chromatography (GC) with flame ionization detection (FID) provides reliable quantification. Method validation should include limits of detection (LOD), recovery rates, and inter-day precision metrics .
Advanced Research Questions
Q. How can structural modifications to this compound be systematically evaluated for their impact on receptor binding or metabolic pathways?
Adopt a structure-activity relationship (SAR) framework combining in silico docking studies (e.g., AutoDock Vina) with in vitro assays. For receptor binding, use competitive radioligand assays (e.g., ³H-labeled ligands) to measure IC₅₀ values. Metabolic pathway analysis requires stable isotope tracing (¹³C or ²H) and hepatocyte incubation followed by metabolite profiling via UPLC-QTOF-MS. Multivariate statistical analysis (PCA or PLS-DA) identifies key structural determinants of activity .
Q. What experimental strategies resolve contradictions in reported pharmacological data for this compound derivatives?
Contradictions may arise from differences in assay conditions (e.g., cell line variability, solvent effects). Address these by:
- Replicating studies under standardized conditions (e.g., ATCC cell lines, controlled DMSO concentrations ≤0.1%).
- Performing meta-analyses of published IC₅₀ values with sensitivity-weighted statistical models.
- Validating target specificity using CRISPR/Cas9 knockout models or isoform-selective inhibitors .
Q. How can researchers optimize analytical workflows to detect trace this compound metabolites in heterogeneous samples?
Implement a two-phase approach:
Sample Preparation : Solid-phase extraction (SPE) with mixed-mode sorbents to enrich metabolites, followed by derivatization (e.g., silylation for GC-MS compatibility).
Detection : High-resolution mass spectrometry (HRMS) in data-independent acquisition (DIA) mode, paired with ion mobility spectrometry (IMS) to separate isobaric species. Use open-source tools (e.g., XCMS Online) for peak alignment and annotation .
Q. What computational models are effective for predicting this compound’s physicochemical properties and bioactivity?
Leverage quantitative structure-property relationship (QSPR) models using descriptors like logP, polar surface area, and H-bond donor/acceptor counts. For bioactivity, machine learning platforms (e.g., Chemprop) trained on ChEMBL datasets predict target engagement. Cross-validate predictions with experimental ADME assays (e.g., Caco-2 permeability, microsomal stability) .
Q. How should researchers design experiments to investigate this compound’s stability under extreme physiological or environmental conditions?
Use accelerated stability testing:
- Thermal Stress : Incubate samples at 40–60°C for 4–8 weeks, monitoring degradation via HPLC-UV.
- Oxidative Stress : Expose to 0.1–3% H₂O₂ and analyze peroxide adducts via LC-MSⁿ.
- Photolytic Stress : Follow ICH Q1B guidelines using a xenon arc lamp. Document degradation kinetics using Arrhenius plots to extrapolate shelf-life .
Q. Methodological Best Practices
- Reproducibility : Archive raw spectral data and chromatograms in FAIR-compliant repositories (e.g., Zenodo) .
- Ethical Reporting : Disclose conflicts of interest and adhere to ARRIVE guidelines for in vivo studies .
- Data Contradictions : Use Bayesian statistics to weigh evidence quality and prioritize follow-up experiments .
Eigenschaften
IUPAC Name |
(5S,8R,9S,10S,13R,14S,17R)-10,13-dimethyl-17-[(2R)-pentan-2-yl]-2,3,4,5,6,7,8,9,11,12,14,15,16,17-tetradecahydro-1H-cyclopenta[a]phenanthrene | |
---|---|---|
Source | PubChem | |
URL | https://pubchem.ncbi.nlm.nih.gov | |
Description | Data deposited in or computed by PubChem | |
InChI |
InChI=1S/C24H42/c1-5-8-17(2)20-12-13-21-19-11-10-18-9-6-7-15-23(18,3)22(19)14-16-24(20,21)4/h17-22H,5-16H2,1-4H3/t17-,18+,19+,20-,21+,22+,23+,24-/m1/s1 | |
Source | PubChem | |
URL | https://pubchem.ncbi.nlm.nih.gov | |
Description | Data deposited in or computed by PubChem | |
InChI Key |
QSHQKIURKJITMZ-OBUPQJQESA-N | |
Source | PubChem | |
URL | https://pubchem.ncbi.nlm.nih.gov | |
Description | Data deposited in or computed by PubChem | |
Canonical SMILES |
CCCC(C)C1CCC2C1(CCC3C2CCC4C3(CCCC4)C)C | |
Source | PubChem | |
URL | https://pubchem.ncbi.nlm.nih.gov | |
Description | Data deposited in or computed by PubChem | |
Isomeric SMILES |
CCC[C@@H](C)[C@H]1CC[C@@H]2[C@@]1(CC[C@H]3[C@H]2CC[C@H]4[C@@]3(CCCC4)C)C | |
Source | PubChem | |
URL | https://pubchem.ncbi.nlm.nih.gov | |
Description | Data deposited in or computed by PubChem | |
Molecular Formula |
C24H42 | |
Source | PubChem | |
URL | https://pubchem.ncbi.nlm.nih.gov | |
Description | Data deposited in or computed by PubChem | |
Molecular Weight |
330.6 g/mol | |
Source | PubChem | |
URL | https://pubchem.ncbi.nlm.nih.gov | |
Description | Data deposited in or computed by PubChem | |
CAS No. |
80373-86-0 | |
Record name | Cholane | |
Source | ChemIDplus | |
URL | https://pubchem.ncbi.nlm.nih.gov/substance/?source=chemidplus&sourceid=0080373860 | |
Description | ChemIDplus is a free, web search system that provides access to the structure and nomenclature authority files used for the identification of chemical substances cited in National Library of Medicine (NLM) databases, including the TOXNET system. | |
Record name | CHOLANE | |
Source | FDA Global Substance Registration System (GSRS) | |
URL | https://gsrs.ncats.nih.gov/ginas/app/beta/substances/6EF0441D4P | |
Description | The FDA Global Substance Registration System (GSRS) enables the efficient and accurate exchange of information on what substances are in regulated products. Instead of relying on names, which vary across regulatory domains, countries, and regions, the GSRS knowledge base makes it possible for substances to be defined by standardized, scientific descriptions. | |
Explanation | Unless otherwise noted, the contents of the FDA website (www.fda.gov), both text and graphics, are not copyrighted. They are in the public domain and may be republished, reprinted and otherwise used freely by anyone without the need to obtain permission from FDA. Credit to the U.S. Food and Drug Administration as the source is appreciated but not required. | |
Retrosynthesis Analysis
AI-Powered Synthesis Planning: Our tool employs the Template_relevance Pistachio, Template_relevance Bkms_metabolic, Template_relevance Pistachio_ringbreaker, Template_relevance Reaxys, Template_relevance Reaxys_biocatalysis model, leveraging a vast database of chemical reactions to predict feasible synthetic routes.
One-Step Synthesis Focus: Specifically designed for one-step synthesis, it provides concise and direct routes for your target compounds, streamlining the synthesis process.
Accurate Predictions: Utilizing the extensive PISTACHIO, BKMS_METABOLIC, PISTACHIO_RINGBREAKER, REAXYS, REAXYS_BIOCATALYSIS database, our tool offers high-accuracy predictions, reflecting the latest in chemical research and data.
Strategy Settings
Precursor scoring | Relevance Heuristic |
---|---|
Min. plausibility | 0.01 |
Model | Template_relevance |
Template Set | Pistachio/Bkms_metabolic/Pistachio_ringbreaker/Reaxys/Reaxys_biocatalysis |
Top-N result to add to graph | 6 |
Feasible Synthetic Routes
Haftungsausschluss und Informationen zu In-Vitro-Forschungsprodukten
Bitte beachten Sie, dass alle Artikel und Produktinformationen, die auf BenchChem präsentiert werden, ausschließlich zu Informationszwecken bestimmt sind. Die auf BenchChem zum Kauf angebotenen Produkte sind speziell für In-vitro-Studien konzipiert, die außerhalb lebender Organismen durchgeführt werden. In-vitro-Studien, abgeleitet von dem lateinischen Begriff "in Glas", beinhalten Experimente, die in kontrollierten Laborumgebungen unter Verwendung von Zellen oder Geweben durchgeführt werden. Es ist wichtig zu beachten, dass diese Produkte nicht als Arzneimittel oder Medikamente eingestuft sind und keine Zulassung der FDA für die Vorbeugung, Behandlung oder Heilung von medizinischen Zuständen, Beschwerden oder Krankheiten erhalten haben. Wir müssen betonen, dass jede Form der körperlichen Einführung dieser Produkte in Menschen oder Tiere gesetzlich strikt untersagt ist. Es ist unerlässlich, sich an diese Richtlinien zu halten, um die Einhaltung rechtlicher und ethischer Standards in Forschung und Experiment zu gewährleisten.