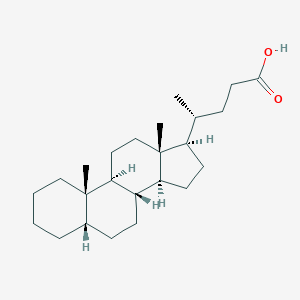
5beta-Cholanic acid
Übersicht
Beschreibung
5β-Cholanic acid is a bile acid derivative and a core structure for many steroidal compounds. Its chemical formula is C₂₄H₄₀O₃, derived from the cholane skeleton with a carboxyl group at position 24 and a β-configuration at the C5 position . Unlike hydroxylated bile acids (e.g., cholic acid), 5β-cholanic acid lacks hydroxyl groups on its steroid nucleus, making it a foundational structure for synthesizing derivatives with modified biological activities .
Key applications include its role in drug delivery systems, where hydrophobically modified glycol chitosan bearing 5β-cholanic acid forms self-assembled nanoparticles with low critical aggregation concentrations (CACs) . It also acts as a ligand for nuclear receptors like the farnesoid X receptor (FXR), influencing cholesterol homeostasis .
Vorbereitungsmethoden
Oxidation of Chenodeoxycholic Acid Using Hydrogen Peroxide
Reaction Mechanism and Conditions
The oxidation of chenodeoxycholic acid to 3α-hydroxy-7-oxo-5β-cholanic acid is catalyzed by hydrogen peroxide in the presence of an acid catalyst. The reaction proceeds via selective oxidation of the 7α-hydroxyl group to a ketone while retaining the 5β-cholanic acid backbone .
Key Steps :
-
Mixing Phase : Chenodeoxycholic acid is dissolved in an alcoholic solvent (methanol, ethanol, or isopropanol) with an acid catalyst (citric acid, tartaric acid, or sulfuric acid) at a mass ratio of 1:5–10:0.1 (chenodeoxycholic acid:solvent:catalyst) .
-
Heating and Cooling : The mixture is heated to 30–50°C for 30–60 minutes, followed by cooling to 2–5°C to stabilize intermediates .
-
Oxidation : Aqueous hydrogen peroxide (28 wt%) is added dropwise at ≤15°C, followed by a 3–5 hour reaction at 10–12°C .
-
Workup : Methanol is partially evaporated, and water is added to precipitate the crude product, which is filtered, washed, and dried .
Purification Techniques
Crude 3α-hydroxy-7-oxo-5β-cholanic acid is purified using mixed solvents (e.g., isopropanol:ethyl acetate = 1:3 v/v) with activated carbon decolorization. Recrystallization at 2°C yields a refined product with 98.2% purity and 82.5% yield .
Table 1: Optimization of Hydrogen Peroxide Oxidation
Sodium Hypochlorite-Mediated Oxidation
Synthesis of 3α-Hydroxy-7β-Carbonyl-5β-Cholanic Acid
An alternative route employs sodium hypochlorite under acidic conditions to oxidize chenodeoxycholic acid. This method generates 3α-hydroxy-7β-carbonyl-5β-cholanic acid, a precursor for ursodeoxycholic acid .
Procedure :
-
Oxidation : Chenodeoxycholic acid reacts with sodium hypochlorite in an acidic medium (e.g., HCl), forming hypochlorous acid in situ.
-
Reduction : The intermediate is hydrogenated using Raney nickel to yield ursodeoxycholic acid, but the 5β configuration remains intact during oxidation .
Advantages :
-
High selectivity for the 7β-ketone group.
-
Shorter reaction time compared to hydrogen peroxide methods .
Comparative Analysis of Methods
Table 2: Method Comparison for 5β-Cholanic Acid Derivatives
Analyse Chemischer Reaktionen
Types of Reactions: Ursocholanic acid undergoes various chemical reactions, including oxidation, reduction, and substitution reactions. These reactions are essential for modifying the compound’s structure and enhancing its biological activity.
Common Reagents and Conditions: Common reagents used in the reactions of ursocholanic acid include oxidizing agents like potassium permanganate and reducing agents such as sodium borohydride. The reactions are typically carried out under controlled conditions to ensure the desired product formation.
Major Products Formed: The major products formed from the reactions of ursocholanic acid include derivatives with enhanced biological activity. For example, oxidation of ursocholanic acid can lead to the formation of ursodeoxycholic acid, which has significant therapeutic applications .
Wissenschaftliche Forschungsanwendungen
1.1. Bile Acids as Therapeutic Agents
Bile acids, including 5beta-Cholanic acid, have been studied for their potential therapeutic roles in various diseases. They have shown promise in treating conditions such as:
- Primary Biliary Cirrhosis (PBC) : Ursodeoxycholic acid, a bile acid, is FDA-approved for PBC treatment, highlighting the therapeutic potential of bile acids .
- Cancer Treatment : Research indicates that bile acids can enhance the sensitivity of cancer cells to chemotherapeutic agents like doxorubicin. Specifically, this compound has been shown to inhibit ABCC1-mediated drug transport, thereby increasing doxorubicin accumulation in resistant tumor cells .
1.2. Activation of Nuclear Receptors
This compound derivatives have been identified as potent activators of the farnesoid X receptor (FXR). FXR plays a crucial role in regulating cholesterol and lipid metabolism. The activation of FXR by these derivatives can lead to:
- Hypolipidemic Effects : Studies have demonstrated that certain derivatives can induce the expression of FXR target genes in liver cells, promoting lipid regulation and potentially serving as pharmaceutical agents for cholesterol-related diseases .
2.1. Enzymatic Reactions
This compound serves as a substrate for various enzymes involved in lipid metabolism. Its derivatives are utilized in enzymatic reactions that facilitate:
- Bile Salt Formation : The conversion of this compound into bile salts through conjugation with amino acids like glycine or taurine plays a vital role in emulsifying dietary fats and aiding absorption in the intestines .
3.1. Enhancing Drug Delivery
Recent studies have focused on the role of bile acids in improving drug delivery systems:
- A study demonstrated that the incorporation of this compound into drug formulations could enhance bioavailability and therapeutic efficacy due to its ability to modulate membrane permeability and transport mechanisms .
3.2. Clinical Investigations
Clinical trials are underway to further explore the therapeutic applications of this compound derivatives:
Wirkmechanismus
Ursocholanic acid exerts its effects through several mechanisms. It activates the glucocorticoid receptor, leading to increased phosphorylation of Akt and improved mitochondrial function . This activation helps in maintaining mitochondrial integrity and cellular signaling, which is crucial for its therapeutic effects.
Vergleich Mit ähnlichen Verbindungen
Comparison with Structurally Similar Compounds
Structural and Functional Differences
The biological and physicochemical properties of 5β-cholanic acid derivatives depend on the number, position, and type of substituents (e.g., hydroxyl, keto, or acetyloxy groups). Below is a comparative analysis:
Table 1: Key Structural and Functional Differences
Physicochemical Properties
- Self-Assembly Behavior: Derivatives like hydrophobically modified glycol chitosan (HGC) with 5β-cholanic acid form spherical nanoparticles. The CAC decreases with higher degrees of substitution (DS), enabling compact hydrophobic cores .
- Solubility : Hydroxyl groups (e.g., in cholic acid) increase hydrophilicity, while keto or acetyloxy groups (e.g., 7-keto deoxycholic acid) enhance lipophilicity .
Drug Delivery Systems
5β-Cholanic acid-based nanoparticles (e.g., HGCs) exhibit tunable sizes (50–200 nm) and low CACs (0.01–0.1 mg/mL), making them ideal for encapsulating hydrophobic drugs .
Therapeutic Potential
- FXR-Targeted Therapies: 5β-Cholanic acid derivatives are being optimized for treating metabolic disorders. For example, marchantin A (a non-steroidal FXR activator) shares functional similarities with bile acids .
Biologische Aktivität
5beta-Cholanic acid, a bile acid derivative, has garnered attention for its diverse biological activities, particularly in the context of metabolism and potential therapeutic applications. This article explores the compound's biological activity, focusing on its mechanisms of action, clinical implications, and recent research findings.
Overview of this compound
This compound is a steroid compound derived from cholesterol metabolism. It is a precursor to various bile acids that play crucial roles in lipid digestion and absorption. Bile acids, including this compound derivatives, are known to act as signaling molecules influencing metabolic pathways through their interactions with nuclear receptors such as the farnesoid X receptor (FXR).
Activation of Farnesoid X Receptor (FXR)
FXR is a nuclear receptor that regulates bile acid homeostasis and lipid metabolism. Research indicates that this compound and its derivatives can activate FXR more potently than traditional hydroxylated bile acids. For instance, N-methyl-5beta-glycocholanic acid (NMGCA), a derivative of this compound, has been shown to induce the formation of receptor/coactivator complexes, promoting the expression of FXR target genes in liver cells . This activation leads to significant hypolipidemic effects, suggesting potential applications in treating cholesterol-related disorders.
Biological Activities
-
Hypoglycemic Effects
Studies have highlighted the hypoglycemic properties of certain this compound derivatives, particularly 7α,12α-dihydroxy−12–keto−5β-cholanic acid. This compound has been examined for its potential in managing diabetes mellitus by enhancing insulin sensitivity and glucose metabolism . -
Antibacterial Properties
Bile acids exhibit antibacterial activity, influencing gut microbiota composition. The amphiphilic nature of bile acids allows them to disrupt bacterial membranes, thereby playing a role in maintaining intestinal health and preventing infections . -
Influence on Cholesterol Metabolism
The administration of this compound derivatives has been linked to reduced biliary cholesterol saturation and enhanced cholesterol excretion. This property is particularly significant in gallstone dissolution therapies where bile acids facilitate the solubilization of cholesterol .
Clinical Applications
Therapeutic Potential in Liver Diseases
this compound and its derivatives are being explored for their therapeutic roles in conditions such as primary biliary cholangitis (PBC) and nonalcoholic fatty liver disease (NAFLD). For instance, ursodeoxycholic acid (UDCA), derived from bile acids, is already a standard treatment for PBC due to its ability to improve liver function parameters .
Case Studies
Several clinical trials have investigated the efficacy of bile acid therapy in managing metabolic disorders:
- A study demonstrated that patients receiving UDCA showed improvements in biochemical markers associated with cholestasis during pregnancy .
- Another trial indicated that NMGCA significantly reduced serum cholesterol levels in hyperlipidemic rat models, supporting its potential use as a therapeutic agent for lipid disorders .
Research Findings
Recent studies have focused on synthesizing novel bile acid analogs to enhance their biological activity and therapeutic efficacy. For instance:
- Synthesis and Evaluation : Novel gut-restricted bile acid analogs have been developed to target specific gastrointestinal diseases while minimizing systemic effects .
- Mechanistic Insights : Investigations into the molecular pathways activated by this compound derivatives have revealed their roles in modulating inflammatory responses and metabolic processes .
Summary Table of Biological Activities
Q & A
Basic Research Questions
Q. What standardized analytical techniques are recommended for characterizing the purity and structural integrity of 5beta-Cholanic acid and its derivatives?
- Methodological Answer : Nuclear Magnetic Resonance (NMR) spectroscopy and mass spectrometry (MS) are essential for confirming structural identity, particularly for distinguishing stereoisomers like 3α,7α-dihydroxy derivatives (e.g., chenodeoxycholic acid) . High-Performance Liquid Chromatography (HPLC) with UV/Vis or evaporative light scattering detection is critical for assessing purity, especially when synthesizing deuterated analogs (e.g., 2,2,4,4-D₄-labeled derivatives) . For novel derivatives, elemental analysis or X-ray crystallography may be required to validate molecular composition .
Q. How can researchers ensure reproducibility in synthesizing this compound derivatives?
- Methodological Answer : Detailed protocols must include reaction conditions (temperature, solvent systems, catalysts), purification steps (e.g., recrystallization solvents, column chromatography parameters), and characterization data (Rf values, melting points). For example, deuterated analogs require strict anhydrous conditions and isotopic purity verification via MS . Referencing established guidelines for experimental documentation (e.g., Beilstein Journal of Organic Chemistry) ensures transparency, with supplementary materials providing step-by-step workflows .
Q. What are the key considerations for handling and storing this compound to maintain stability?
- Methodological Answer : Storage at -20°C in airtight, light-resistant containers prevents degradation of labile hydroxyl and ketone groups (e.g., 7-keto derivatives) . Solubility in polar solvents like methanol or dimethyl sulfoxide (DMSO) should be documented, with pH stability tested across physiological ranges (4–8) to simulate in vivo conditions. Lyophilization is recommended for long-term storage of sodium salts (e.g., taurodeoxycholic acid sodium salt) .
Advanced Research Questions
Q. How can researchers resolve discrepancies in reported biological activities of this compound across in vitro and in vivo studies?
- Methodological Answer : Conduct a systematic review with meta-analysis, evaluating variables such as cell line specificity (e.g., hepatocytes vs. cancer cells), pharmacokinetic parameters (e.g., bile acid transporters), and dosage regimes . Use heterogeneity tests (e.g., I² statistic) to identify confounding factors, such as interspecies differences in receptor affinity or metabolic pathways. Weighted analyses should prioritize studies with rigorous blinding and controlled confounding variables .
Q. What experimental design strategies optimize the use of this compound in nanoparticle-based drug delivery systems?
- Methodological Answer : For self-assembled nanoparticles, adjust the molar ratio of this compound to polymers (e.g., glycol chitosan) to balance hydrophobicity and drug-loading capacity. Dynamic light scattering (DLS) and transmission electron microscopy (TEM) are critical for assessing particle size and stability . In vivo studies should incorporate fluorescence or radiolabeling to track biodistribution, with control groups testing unmodified nanoparticles to isolate the contribution of bile acid moieties to targeting efficiency .
Q. How can computational modeling enhance the understanding of this compound’s interactions with nuclear receptors (e.g., FXR, TGR5)?
- Methodological Answer : Molecular docking simulations (using software like AutoDock Vina) can predict binding affinities to receptor pockets, guided by crystallographic data of homologous bile acids. Validate predictions with surface plasmon resonance (SPR) to measure kinetic constants (Ka/Kd) and compare with experimental IC₅₀ values from competitive binding assays. Address discrepancies by refining force field parameters or incorporating solvent effects in simulations .
Q. What methodologies are effective for analyzing the metabolic fate of this compound in complex biological matrices?
- Methodological Answer : Liquid chromatography-tandem mass spectrometry (LC-MS/MS) with multiple reaction monitoring (MRM) enables quantification of phase I/II metabolites (e.g., sulfated or glucuronidated forms) in serum or fecal samples. Isotope dilution assays using ¹³C-labeled internal standards improve accuracy. For pathway analysis, integrate metabolomics datasets with gene expression profiling (e.g., CYP7A1, CYP8B1) to correlate metabolic flux with enzymatic activity .
Q. Guidelines for Addressing Contradictions and Ensuring Rigor
- Data Contradiction Analysis : Apply the PRISMA framework to systematically evaluate conflicting results, emphasizing studies with validated analytical methods (e.g., ISO-certified protocols) .
- Ethical and Reproducibility Standards : Adhere to FAIR data principles (Findable, Accessible, Interoperable, Reusable) by depositing raw datasets in public repositories (e.g., Zenodo) alongside detailed metadata .
Eigenschaften
IUPAC Name |
(4R)-4-[(5S,8R,9S,10S,13R,14S,17R)-10,13-dimethyl-2,3,4,5,6,7,8,9,11,12,14,15,16,17-tetradecahydro-1H-cyclopenta[a]phenanthren-17-yl]pentanoic acid | |
---|---|---|
Source | PubChem | |
URL | https://pubchem.ncbi.nlm.nih.gov | |
Description | Data deposited in or computed by PubChem | |
InChI |
InChI=1S/C24H40O2/c1-16(7-12-22(25)26)19-10-11-20-18-9-8-17-6-4-5-14-23(17,2)21(18)13-15-24(19,20)3/h16-21H,4-15H2,1-3H3,(H,25,26)/t16-,17+,18+,19-,20+,21+,23+,24-/m1/s1 | |
Source | PubChem | |
URL | https://pubchem.ncbi.nlm.nih.gov | |
Description | Data deposited in or computed by PubChem | |
InChI Key |
RPKLZQLYODPWTM-LVVAJZGHSA-N | |
Source | PubChem | |
URL | https://pubchem.ncbi.nlm.nih.gov | |
Description | Data deposited in or computed by PubChem | |
Canonical SMILES |
CC(CCC(=O)O)C1CCC2C1(CCC3C2CCC4C3(CCCC4)C)C | |
Source | PubChem | |
URL | https://pubchem.ncbi.nlm.nih.gov | |
Description | Data deposited in or computed by PubChem | |
Isomeric SMILES |
C[C@H](CCC(=O)O)[C@H]1CC[C@@H]2[C@@]1(CC[C@H]3[C@H]2CC[C@H]4[C@@]3(CCCC4)C)C | |
Source | PubChem | |
URL | https://pubchem.ncbi.nlm.nih.gov | |
Description | Data deposited in or computed by PubChem | |
Molecular Formula |
C24H40O2 | |
Source | PubChem | |
URL | https://pubchem.ncbi.nlm.nih.gov | |
Description | Data deposited in or computed by PubChem | |
DSSTOX Substance ID |
DTXSID501021288 | |
Record name | 5beta-Cholan-24-oic acid | |
Source | EPA DSSTox | |
URL | https://comptox.epa.gov/dashboard/DTXSID501021288 | |
Description | DSSTox provides a high quality public chemistry resource for supporting improved predictive toxicology. | |
Molecular Weight |
360.6 g/mol | |
Source | PubChem | |
URL | https://pubchem.ncbi.nlm.nih.gov | |
Description | Data deposited in or computed by PubChem | |
CAS No. |
546-18-9 | |
Record name | 5β-Cholanic acid | |
Source | CAS Common Chemistry | |
URL | https://commonchemistry.cas.org/detail?cas_rn=546-18-9 | |
Description | CAS Common Chemistry is an open community resource for accessing chemical information. Nearly 500,000 chemical substances from CAS REGISTRY cover areas of community interest, including common and frequently regulated chemicals, and those relevant to high school and undergraduate chemistry classes. This chemical information, curated by our expert scientists, is provided in alignment with our mission as a division of the American Chemical Society. | |
Explanation | The data from CAS Common Chemistry is provided under a CC-BY-NC 4.0 license, unless otherwise stated. | |
Record name | Cholanic acid, (5beta)- | |
Source | ChemIDplus | |
URL | https://pubchem.ncbi.nlm.nih.gov/substance/?source=chemidplus&sourceid=0000546189 | |
Description | ChemIDplus is a free, web search system that provides access to the structure and nomenclature authority files used for the identification of chemical substances cited in National Library of Medicine (NLM) databases, including the TOXNET system. | |
Record name | Ursocholanic acid | |
Source | DTP/NCI | |
URL | https://dtp.cancer.gov/dtpstandard/servlet/dwindex?searchtype=NSC&outputformat=html&searchlist=18161 | |
Description | The NCI Development Therapeutics Program (DTP) provides services and resources to the academic and private-sector research communities worldwide to facilitate the discovery and development of new cancer therapeutic agents. | |
Explanation | Unless otherwise indicated, all text within NCI products is free of copyright and may be reused without our permission. Credit the National Cancer Institute as the source. | |
Record name | 5beta-Cholan-24-oic acid | |
Source | EPA DSSTox | |
URL | https://comptox.epa.gov/dashboard/DTXSID501021288 | |
Description | DSSTox provides a high quality public chemistry resource for supporting improved predictive toxicology. | |
Record name | CHOLANIC ACID, (5.BETA.)- | |
Source | FDA Global Substance Registration System (GSRS) | |
URL | https://gsrs.ncats.nih.gov/ginas/app/beta/substances/970EKW2JTO | |
Description | The FDA Global Substance Registration System (GSRS) enables the efficient and accurate exchange of information on what substances are in regulated products. Instead of relying on names, which vary across regulatory domains, countries, and regions, the GSRS knowledge base makes it possible for substances to be defined by standardized, scientific descriptions. | |
Explanation | Unless otherwise noted, the contents of the FDA website (www.fda.gov), both text and graphics, are not copyrighted. They are in the public domain and may be republished, reprinted and otherwise used freely by anyone without the need to obtain permission from FDA. Credit to the U.S. Food and Drug Administration as the source is appreciated but not required. | |
Retrosynthesis Analysis
AI-Powered Synthesis Planning: Our tool employs the Template_relevance Pistachio, Template_relevance Bkms_metabolic, Template_relevance Pistachio_ringbreaker, Template_relevance Reaxys, Template_relevance Reaxys_biocatalysis model, leveraging a vast database of chemical reactions to predict feasible synthetic routes.
One-Step Synthesis Focus: Specifically designed for one-step synthesis, it provides concise and direct routes for your target compounds, streamlining the synthesis process.
Accurate Predictions: Utilizing the extensive PISTACHIO, BKMS_METABOLIC, PISTACHIO_RINGBREAKER, REAXYS, REAXYS_BIOCATALYSIS database, our tool offers high-accuracy predictions, reflecting the latest in chemical research and data.
Strategy Settings
Precursor scoring | Relevance Heuristic |
---|---|
Min. plausibility | 0.01 |
Model | Template_relevance |
Template Set | Pistachio/Bkms_metabolic/Pistachio_ringbreaker/Reaxys/Reaxys_biocatalysis |
Top-N result to add to graph | 6 |
Feasible Synthetic Routes
Haftungsausschluss und Informationen zu In-Vitro-Forschungsprodukten
Bitte beachten Sie, dass alle Artikel und Produktinformationen, die auf BenchChem präsentiert werden, ausschließlich zu Informationszwecken bestimmt sind. Die auf BenchChem zum Kauf angebotenen Produkte sind speziell für In-vitro-Studien konzipiert, die außerhalb lebender Organismen durchgeführt werden. In-vitro-Studien, abgeleitet von dem lateinischen Begriff "in Glas", beinhalten Experimente, die in kontrollierten Laborumgebungen unter Verwendung von Zellen oder Geweben durchgeführt werden. Es ist wichtig zu beachten, dass diese Produkte nicht als Arzneimittel oder Medikamente eingestuft sind und keine Zulassung der FDA für die Vorbeugung, Behandlung oder Heilung von medizinischen Zuständen, Beschwerden oder Krankheiten erhalten haben. Wir müssen betonen, dass jede Form der körperlichen Einführung dieser Produkte in Menschen oder Tiere gesetzlich strikt untersagt ist. Es ist unerlässlich, sich an diese Richtlinien zu halten, um die Einhaltung rechtlicher und ethischer Standards in Forschung und Experiment zu gewährleisten.