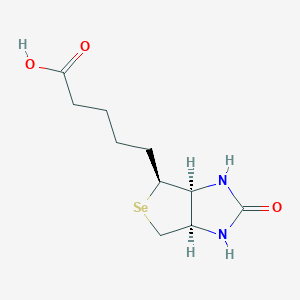
Selenobiotin
Übersicht
Beschreibung
Selenobiotin is a selenium-containing analog of biotin, a vital vitamin that plays a crucial role in various metabolic processes The substitution of sulfur with selenium in the biotin molecule results in this compound, which retains similar biochemical properties but with distinct characteristics due to the presence of selenium
Wissenschaftliche Forschungsanwendungen
Selenobiotin has several scientific research applications, including:
Chemistry: Used as a probe to study selenium biochemistry and its role in enzymatic reactions.
Biology: Investigated for its role in cellular processes and its potential as a selenium supplement.
Medicine: Explored for its antioxidant properties and potential therapeutic applications in diseases related to selenium deficiency.
Industry: Utilized in the development of selenium-enriched products and supplements.
Wirkmechanismus
Target of Action:
Selenobiotin is a novel compound that has garnered attention due to its potential therapeutic applications. One of its primary targets is the biotin receptor (BR) . Biotin receptors are proteins involved in cellular processes, including cell growth, metabolism, and gene expression. This compound specifically interacts with these receptors, which play a crucial role in its mode of action .
Biochemische Analyse
Biochemical Properties
Selenobiotin plays a significant role in biochemical reactions. It interacts with various enzymes, proteins, and other biomolecules. Selenoproteins, which contain one or several selenocysteine residues, are synthesized in the cells via a unique mechanism that involves specific enzymes and factors and directly depends on selenium intake . This compound, being a selenoprotein, is expected to follow similar biochemical pathways.
Cellular Effects
Many selenoproteins have prominent antioxidant properties and can eliminate reactive oxygen species (ROS) formed as byproducts of molecular oxygen reactions in the process of oxidative phosphorylation in the cells . As a selenoprotein, this compound may share these properties.
Molecular Mechanism
It is known that selenoproteins are synthesized in the cells via a unique mechanism that involves specific enzymes and factors and directly depends on selenium intake . This compound, being a selenoprotein, is expected to follow similar molecular mechanisms.
Temporal Effects in Laboratory Settings
A study on selenobiotinyl-streptavidin co-crystal datasets recorded for selenium single-wavelength anomalous diffraction (Se-SAD) structure determination provides some insights .
Dosage Effects in Animal Models
Animal models have played a critical role in exploring and describing disease pathophysiology, identifying targets, and surveying new therapeutic agents and in vivo treatments .
Metabolic Pathways
This compound is likely involved in the metabolic pathways of selenoproteins. Selenoproteins have a wide range of cellular functions including regulation of selenium transport, thyroid hormones, immunity, and redox homeostasis .
Transport and Distribution
Selenoprotein P (Sepp) is known to transport selenium to target tissues . Hepatocytes convert nutritional selenocompounds into Sepp for transport and distribution . As a selenoprotein, this compound might be transported and distributed in a similar manner.
Subcellular Localization
Prediction of subcellular localization of proteins from their amino acid sequences has a long history in bioinformatics and is still actively developing . As a protein, this compound’s subcellular localization could be predicted using similar methods.
Vorbereitungsmethoden
Synthetic Routes and Reaction Conditions: Selenobiotin can be synthesized through the modification of biotin synthase, an enzyme that typically catalyzes the insertion of sulfur into dethiobiotin to form biotin. By substituting sulfur with selenium, this compound is produced. The process involves the reconstitution of the enzyme with iron and selenium to form a [2Fe-2Se] cluster, which facilitates the insertion of selenium into dethiobiotin .
Industrial Production Methods: Industrial production of this compound is not as widespread as biotin, but it follows similar principles. The process involves the use of biotechnological methods to produce the enzyme biotin synthase, which is then modified to incorporate selenium instead of sulfur. This method ensures the efficient production of this compound with high purity.
Analyse Chemischer Reaktionen
Types of Reactions: Selenobiotin undergoes various chemical reactions, including:
Oxidation: this compound can be oxidized to form selenoxide derivatives.
Reduction: It can be reduced back to its original form from selenoxide.
Substitution: Selenium in this compound can be substituted with other chalcogens under specific conditions.
Common Reagents and Conditions:
Oxidation: Hydrogen peroxide or other oxidizing agents can be used.
Reduction: Reducing agents like sodium borohydride are commonly employed.
Substitution: Reactions with sulfur or tellurium compounds under controlled conditions.
Major Products: The major products formed from these reactions include selenoxide derivatives, reduced this compound, and substituted chalcogen compounds.
Vergleich Mit ähnlichen Verbindungen
Biotin: The sulfur analog of selenobiotin, essential for carboxylation reactions.
Selenomethionine: A selenium analog of methionine, used as a dietary supplement and in protein synthesis studies.
Uniqueness: this compound is unique due to its selenium content, which imparts distinct biochemical properties compared to its sulfur analog, biotin. The presence of selenium enhances its antioxidant capabilities and potential therapeutic applications, making it a valuable compound for research and industrial applications.
Biologische Aktivität
Selenobiotin, a selenium-containing derivative of biotin, has garnered attention for its potential biological activities and applications in various fields, including microbiology, nutrition, and cancer research. This article explores the biological activity of this compound, presenting findings from recent studies, case analyses, and relevant data tables to provide a comprehensive overview.
This compound is a compound formed by the incorporation of selenium into the biotin structure. Biotin is a crucial vitamin that serves as a cofactor in various enzymatic reactions, particularly those involved in carbohydrate and fat metabolism. The introduction of selenium into this molecule may enhance its biological properties, making it an interesting subject for research.
Biological Properties
-
Growth Factor Activity :
This compound has been shown to be an effective growth factor for biotin-requiring microorganisms. It supports microbial growth similarly to biotin, indicating its potential utility in microbiological applications . -
Selenium Bioavailability :
The incorporation of selenium into biotin enhances the bioavailability of selenium for organisms that require this micronutrient. This is particularly important as selenium plays critical roles in antioxidant defense and immune function . -
Antioxidant Properties :
Like other selenium compounds, this compound exhibits antioxidant properties that can protect cells from oxidative stress. This is significant in the context of cancer prevention and treatment, as oxidative stress is a known contributor to tumorigenesis .
Case Studies and Experimental Data
-
Microbial Synthesis :
A study demonstrated the biosynthesis of this compound by yeasts such as Meyerozyma guilliermondii and Trichosporon cutaneum. The latter strain produced this compound at concentrations up to 28.4% of the total biotin vitamer fraction when cultured with selenomethionine . -
Selenium Accumulation :
The research highlighted significant differences in selenium accumulation among various yeast strains when supplemented with different forms of selenium. For instance, T. cutaneum yielded biomass with selenium content ranging from 284.134 µg/g DM to 909.900 µg/g DM depending on the selenium source used . -
Antioxidant Activity :
In vitro studies have shown that this compound can induce apoptosis selectively in cancer cells while sparing non-malignant cells, suggesting its potential as an anticancer agent .
Data Tables
Strain | Selenium Source | Biomass Yield (g DM/L) | Selenium Content (µg/g DM) |
---|---|---|---|
Meyerozyma guilliermondii | Selenomethionine | 9.578 ± 0.433 | 188.064 ± 6.935 |
Trichosporon cutaneum | Sodium selenate | 6.116 ± 0.132 | 37.434 ± 3.394 |
Candida utilis | Sodium selenite | 13.35 | 1010 |
The biological activity of this compound is primarily attributed to its role in enhancing the synthesis of selenoproteins—proteins that incorporate selenium as a key component and are essential for various cellular functions including antioxidant defense and regulation of thyroid hormones .
Selenoprotein Interaction
Recent studies have indicated that specific selenoproteins may exhibit dual roles in cancer biology, potentially acting as both tumor suppressors and promoters depending on the cellular context and concentration of selenium present . This complexity necessitates further investigation into how this compound can modulate these pathways effectively.
Future Directions
Given the promising results regarding the biological activity of this compound, future research should focus on:
- Clinical Trials : Evaluating the efficacy of this compound in human populations, particularly concerning cancer prevention and treatment.
- Mechanistic Studies : Elucidating the precise molecular mechanisms through which this compound exerts its effects on cellular processes.
- Microbial Applications : Exploring the use of this compound in enhancing selenium biofortification strategies in crops.
Eigenschaften
IUPAC Name |
5-[(3aS,4S,6aR)-2-oxo-1,3,3a,4,6,6a-hexahydroselenopheno[3,4-d]imidazol-4-yl]pentanoic acid | |
---|---|---|
Source | PubChem | |
URL | https://pubchem.ncbi.nlm.nih.gov | |
Description | Data deposited in or computed by PubChem | |
InChI |
InChI=1S/C10H16N2O3Se/c13-8(14)4-2-1-3-7-9-6(5-16-7)11-10(15)12-9/h6-7,9H,1-5H2,(H,13,14)(H2,11,12,15)/t6-,7-,9-/m0/s1 | |
Source | PubChem | |
URL | https://pubchem.ncbi.nlm.nih.gov | |
Description | Data deposited in or computed by PubChem | |
InChI Key |
HVUDXAKXEONARI-ZKWXMUAHSA-N | |
Source | PubChem | |
URL | https://pubchem.ncbi.nlm.nih.gov | |
Description | Data deposited in or computed by PubChem | |
Canonical SMILES |
C1C2C(C([Se]1)CCCCC(=O)O)NC(=O)N2 | |
Source | PubChem | |
URL | https://pubchem.ncbi.nlm.nih.gov | |
Description | Data deposited in or computed by PubChem | |
Isomeric SMILES |
C1[C@H]2[C@@H]([C@@H]([Se]1)CCCCC(=O)O)NC(=O)N2 | |
Source | PubChem | |
URL | https://pubchem.ncbi.nlm.nih.gov | |
Description | Data deposited in or computed by PubChem | |
Molecular Formula |
C10H16N2O3Se | |
Source | PubChem | |
URL | https://pubchem.ncbi.nlm.nih.gov | |
Description | Data deposited in or computed by PubChem | |
DSSTOX Substance ID |
DTXSID00973528 | |
Record name | 5-(2-Hydroxy-3a,4,6,6a-tetrahydro-1H-selenopheno[3,4-d]imidazol-6-yl)pentanoic acid | |
Source | EPA DSSTox | |
URL | https://comptox.epa.gov/dashboard/DTXSID00973528 | |
Description | DSSTox provides a high quality public chemistry resource for supporting improved predictive toxicology. | |
Molecular Weight |
291.22 g/mol | |
Source | PubChem | |
URL | https://pubchem.ncbi.nlm.nih.gov | |
Description | Data deposited in or computed by PubChem | |
CAS No. |
57956-29-3 | |
Record name | Selenobiotin | |
Source | ChemIDplus | |
URL | https://pubchem.ncbi.nlm.nih.gov/substance/?source=chemidplus&sourceid=0057956293 | |
Description | ChemIDplus is a free, web search system that provides access to the structure and nomenclature authority files used for the identification of chemical substances cited in National Library of Medicine (NLM) databases, including the TOXNET system. | |
Record name | 5-(2-Hydroxy-3a,4,6,6a-tetrahydro-1H-selenopheno[3,4-d]imidazol-6-yl)pentanoic acid | |
Source | EPA DSSTox | |
URL | https://comptox.epa.gov/dashboard/DTXSID00973528 | |
Description | DSSTox provides a high quality public chemistry resource for supporting improved predictive toxicology. | |
Retrosynthesis Analysis
AI-Powered Synthesis Planning: Our tool employs the Template_relevance Pistachio, Template_relevance Bkms_metabolic, Template_relevance Pistachio_ringbreaker, Template_relevance Reaxys, Template_relevance Reaxys_biocatalysis model, leveraging a vast database of chemical reactions to predict feasible synthetic routes.
One-Step Synthesis Focus: Specifically designed for one-step synthesis, it provides concise and direct routes for your target compounds, streamlining the synthesis process.
Accurate Predictions: Utilizing the extensive PISTACHIO, BKMS_METABOLIC, PISTACHIO_RINGBREAKER, REAXYS, REAXYS_BIOCATALYSIS database, our tool offers high-accuracy predictions, reflecting the latest in chemical research and data.
Strategy Settings
Precursor scoring | Relevance Heuristic |
---|---|
Min. plausibility | 0.01 |
Model | Template_relevance |
Template Set | Pistachio/Bkms_metabolic/Pistachio_ringbreaker/Reaxys/Reaxys_biocatalysis |
Top-N result to add to graph | 6 |
Feasible Synthetic Routes
Q1: What is the primary evidence suggesting selenobiotin can be biologically synthesized?
A1: Research indicates that this compound, the selenium analog of biotin, can be produced enzymatically. A study successfully demonstrated the production of this compound by depleting the iron-sulfur cluster from Escherichia coli biotin synthase and reconstituting it with iron and selenium. [] This modified enzyme, containing a [2Fe-2Se]2+ cluster, catalyzed the insertion of selenium into dethiobiotin, forming this compound. This finding provides strong evidence for the potential of biological this compound biosynthesis. []
Q2: How does the activity of the [2Fe-2Se]2+ biotin synthase compare to the native enzyme?
A2: While the [2Fe-2Se]2+ biotin synthase can produce this compound, its activity is lower compared to the native [2Fe-2S]2+ enzyme utilizing sulfur. [] Interestingly, when the [2Fe-2Se]2+ enzyme is assayed with Na2S, leading to a [4Fe-4S]2+ cluster, the activity improves, and a mixture of biotin and this compound is produced. [] This suggests a degree of chalcogen exchange within the cluster during the reaction, further highlighting the complexity of the catalytic mechanism.
Q3: What structural insights do we have regarding this compound and its interactions?
A3: While a full structural characterization is still pending, there's information about this compound's interaction with streptavidin, a protein known for its high affinity towards biotin. Research mentions an "SFX structure of corestreptavidin-selenobiotin complex". [] This suggests that, similar to biotin, this compound can also bind to streptavidin, possibly through a similar mechanism. Further research is needed to elucidate the specifics of this interaction and its potential implications.
Q4: Are there synthetic routes available for producing this compound?
A5: Yes, a total synthesis of this compound has been achieved. [] Additionally, a separate study focuses on the "SYNTHESIS OF (+)-SELENOBIOTIN". [] These synthetic approaches are essential for obtaining sufficient quantities of this compound for further research and potential applications.
Haftungsausschluss und Informationen zu In-Vitro-Forschungsprodukten
Bitte beachten Sie, dass alle Artikel und Produktinformationen, die auf BenchChem präsentiert werden, ausschließlich zu Informationszwecken bestimmt sind. Die auf BenchChem zum Kauf angebotenen Produkte sind speziell für In-vitro-Studien konzipiert, die außerhalb lebender Organismen durchgeführt werden. In-vitro-Studien, abgeleitet von dem lateinischen Begriff "in Glas", beinhalten Experimente, die in kontrollierten Laborumgebungen unter Verwendung von Zellen oder Geweben durchgeführt werden. Es ist wichtig zu beachten, dass diese Produkte nicht als Arzneimittel oder Medikamente eingestuft sind und keine Zulassung der FDA für die Vorbeugung, Behandlung oder Heilung von medizinischen Zuständen, Beschwerden oder Krankheiten erhalten haben. Wir müssen betonen, dass jede Form der körperlichen Einführung dieser Produkte in Menschen oder Tiere gesetzlich strikt untersagt ist. Es ist unerlässlich, sich an diese Richtlinien zu halten, um die Einhaltung rechtlicher und ethischer Standards in Forschung und Experiment zu gewährleisten.