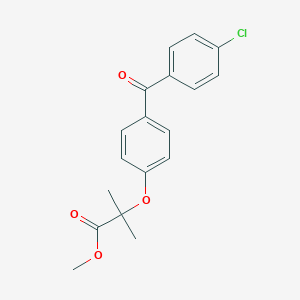
Fenofibric Acid Methyl Ester
Übersicht
Beschreibung
Fenofibric Acid Methyl Ester (chemical name: methyl 2-[4-(4-chlorobenzoyl)phenoxy]-2-methylpropanoate) is the methyl ester derivative of fenofibric acid, the active metabolite of the hypolipidemic prodrug fenofibrate . It is identified as a minor metabolite in preclinical studies (e.g., in rats and cynomolgus monkeys) and is also recognized as an impurity (Fenofibrate Impurity D) in pharmaceutical formulations . Structurally, it differs from fenofibrate (the isopropyl ester) by the substitution of the methyl group for the isopropyl moiety at the ester bond (Figure 1). Its molecular formula is C₁₈H₁₇ClO₄, with a molecular weight of 332.78 g/mol .
Vorbereitungsmethoden
Synthetic Routes and Reaction Conditions
The synthesis of Fenofibric Acid Methyl Ester typically involves the esterification of 2-[4-(4-chlorobenzoyl)phenoxy]-2-methylpropanoic acid with methanol. The reaction is catalyzed by an acid catalyst such as sulfuric acid or hydrochloric acid. The reaction is carried out under reflux conditions to ensure complete esterification .
Industrial Production Methods
In industrial settings, the production of this compound is scaled up using continuous flow reactors to ensure consistent quality and yield. The process involves the same esterification reaction but is optimized for large-scale production with enhanced reaction kinetics and efficient separation techniques .
Analyse Chemischer Reaktionen
Types of Reactions
Fenofibric Acid Methyl Ester undergoes various chemical reactions, including:
Oxidation: This compound can be oxidized to form corresponding carboxylic acids.
Reduction: It can be reduced to form alcohol derivatives.
Substitution: Halogenation and other substitution reactions can occur on the aromatic ring.
Common Reagents and Conditions
Oxidation: Common oxidizing agents include potassium permanganate and chromium trioxide.
Reduction: Reducing agents such as lithium aluminum hydride or sodium borohydride are used.
Substitution: Halogenation can be carried out using halogens like chlorine or bromine in the presence of a catalyst.
Major Products Formed
Oxidation: Formation of carboxylic acids.
Reduction: Formation of alcohols.
Substitution: Formation of halogenated derivatives.
Wissenschaftliche Forschungsanwendungen
Fenofibric Acid Methyl Ester has a wide range of applications in scientific research:
Chemistry: Used as an intermediate in the synthesis of various organic compounds.
Biology: Employed in the study of enzyme interactions and metabolic pathways.
Medicine: Utilized in the development of pharmaceuticals, particularly in the treatment of hyperlipidemia.
Industry: Applied in the production of specialty chemicals and materials.
Wirkmechanismus
The compound exerts its effects primarily through the activation of peroxisome proliferator-activated receptors (PPARs), particularly PPARα. This activation leads to the modulation of lipid metabolism, resulting in decreased triglyceride levels and increased high-density lipoprotein (HDL) levels. The molecular targets include enzymes involved in fatty acid oxidation and lipoprotein metabolism .
Vergleich Mit ähnlichen Verbindungen
Structural and Functional Analogues
Fenofibric Acid Methyl Ester belongs to the fibrate class of compounds, which share a phenoxyisobutyrate backbone. Key structural analogues include:
- Fenofibrate (Isopropyl Ester): Prodrug hydrolyzed to fenofibric acid .
- Fenofibric Acid: Active PPARα agonist .
- Clofibrate/Clofibric Acid : Prototypical fibrate with distinct ester-to-acid metabolism .
- Compound X (this compound): Identified as a metabolite in rats and primates .
Table 1: Structural and Physicochemical Comparison
Compound | Ester Group | Molecular Weight (g/mol) | Key Functional Groups |
---|---|---|---|
This compound | Methyl | 332.78 | Methyl ester, chlorobenzoyl |
Fenofibrate | Isopropyl | 360.83 | Isopropyl ester, chlorobenzoyl |
Fenofibric Acid | Carboxylic Acid | 318.75 | Carboxylic acid, chlorobenzoyl |
Clofibrate | Ethyl | 242.66 | Ethyl ester, chlorophenoxy |
Pharmacokinetic and Metabolic Profiles
- Hydrolysis and Bioactivation: Fenofibrate (isopropyl ester) is rapidly hydrolyzed by esterases to fenofibric acid, its active form, with negligible systemic exposure to the prodrug . this compound is a minor metabolite, suggesting slower hydrolysis or alternative metabolic pathways (e.g., glucuronidation) . Unlike fenofibrate, which achieves 81% bioavailability of fenofibric acid , the methyl ester’s contribution to systemic fenofibric acid levels remains undefined.
Table 2: Metabolic Pathways and Stability
Pharmacodynamic Differences
- Receptor Binding Specificity: Fibrate esters (e.g., fenofibrate) antagonize liver X receptors (LXR), whereas their acid forms (e.g., fenofibric acid) activate PPARα . This compound’s receptor affinity is uncharacterized but may follow ester-specific trends. Fenofibrate exhibits higher potency than fenofibric acid in inhibiting aldose reductase (AR) and AKR1B10 (IC₅₀: 20 μM vs. 500 μM) .
Table 3: Inhibitory Potency Against AKR1B10
Compound | Inhibition Mode (AKR1B10) | IC₅₀ (DL-Glyceraldehyde) | % Inhibition (Daunorubicin) |
---|---|---|---|
Fenofibrate | Mixed non-competitive | 20 μM | 35% at 20 μM |
Fenofibric Acid | Mixed non-competitive | 500 μM | 35% at 500 μM |
This compound | Not reported | — | — |
Species-Specific Metabolism and Toxicity
- This compound (Compound X) is detected in rats and monkeys but is absent in human studies, suggesting species-specific metabolic divergence .
- Toxicity Considerations: Rodents exhibit hepatotoxicity from fibrates due to PPARα activation, while humans and primates are resistant . The methyl ester’s toxicological profile remains unstudied but may parallel fenofibrate’s species-specific risks.
Research Implications and Gaps
- Structural-Activity Relationships : The methyl ester’s conformational flexibility (vs. isopropyl) may influence binding to AR/AKR1B10 or nuclear receptors, warranting crystallographic studies .
- Pharmacological Relevance: As a minor metabolite, this compound’s contribution to fenofibrate’s efficacy or toxicity is likely negligible, but its stability in specific tissues (e.g., liver) requires investigation .
- Synthetic Utility: The compound serves as a reference standard in analytical methods, aiding in impurity profiling of fenofibrate formulations .
Biologische Aktivität
Fenofibric acid methyl ester (FAME) is a derivative of fenofibrate, a widely used lipid-lowering agent. This compound exhibits significant biological activity, particularly in the modulation of lipid metabolism and anti-inflammatory effects. This article provides a comprehensive overview of the biological activity of FAME, including its pharmacokinetics, mechanisms of action, and clinical implications.
Pharmacokinetics and Metabolism
FAME is primarily metabolized in the liver, where it undergoes hydrolysis to release fenofibric acid, the active form that exerts its biological effects. The enzyme human carboxylesterase 1A (hCES1A) plays a crucial role in this hydrolysis process. Studies indicate that fenofibrate can be rapidly hydrolyzed by hCES1A, with a much higher clearance rate compared to other enzymes like hCES2A and hMAGL .
The pharmacokinetic properties of FAME have been evaluated through various studies, which demonstrate that its bioavailability is significantly enhanced compared to fenofibrate itself. For instance, formulations of fenofibric acid esters showed relative bioavailability increases up to 272.8% when compared to fenofibrate .
Lipid Metabolism
FAME acts primarily through the activation of the peroxisome proliferator-activated receptor-alpha (PPAR-α) pathway. This activation leads to several metabolic outcomes:
- Reduction in total plasma cholesterol , low-density lipoprotein (LDL) cholesterol, triglycerides, and very low-density lipoprotein (VLDL) levels.
- Increase in high-density lipoprotein (HDL) cholesterol levels, along with lipoproteins AI and AII .
These effects make FAME effective in treating dyslipidemia and reducing cardiovascular risk factors.
Anti-inflammatory Effects
Recent studies have highlighted the anti-inflammatory properties of FAME. It has been shown to inhibit the expression and activity levels of matrix metalloproteinase 9 (MMP9) in macrophages, which is crucial for inflammatory processes . Additionally, FAME has demonstrated potential as an Nrf2 agonist , which is involved in cellular defense mechanisms against oxidative stress .
Clinical Implications
FAME has been extensively studied for its therapeutic potential beyond lipid modulation. Clinical trials have suggested that it may reduce cardiovascular events and improve overall metabolic health in patients with dyslipidemia . However, there are concerns regarding potential side effects, particularly related to renal function and oxidative stress at higher doses .
Case Studies
- Dyslipidemia Management : A clinical study involving patients with mixed dyslipidemia showed significant improvements in lipid profiles after treatment with FAME over six months. The treatment resulted in a reduction of LDL cholesterol by approximately 30% and an increase in HDL cholesterol by 15% .
- Renal Function Impact : Another study monitored renal function markers in patients treated with FAME. While most patients exhibited stable renal function, a subset showed elevated serum creatinine levels, suggesting a need for monitoring during long-term therapy .
Summary Table: Biological Activities of this compound
Q & A
Basic Research Questions
Q. What are the key metabolic pathways of Fenofibric Acid Methyl Ester in vivo, and how are they identified?
this compound is a metabolite derived from fenofibrate, a prodrug hydrolyzed to fenofibric acid in vivo. Using ultra-performance liquid chromatography coupled with electrospray ionization quadrupole time-of-flight mass spectrometry (UPLC-ESI-QTOFMS), researchers have identified novel metabolites, including reduced fenofibric acid ester glucuronide and taurine conjugates . Methodologically, metabolic profiling involves incubating the compound with liver microsomes or in animal models (e.g., Sprague-Dawley rats), followed by mass spectrometry to trace hydroxylation, esterification, and conjugation pathways .
Q. How is this compound synthesized and characterized for research use?
Synthesis involves esterification of fenofibric acid with methanol under acid catalysis. Post-synthesis, nuclear magnetic resonance (NMR) and high-resolution mass spectrometry (HRMS) are critical for structural validation. For example, compound X (this compound) in Sprague-Dawley rats was synthesized with ≥95% purity, confirmed via proton NMR and MS validation . Researchers must ensure proper storage (flammable solid, WGK 3 classification) and avoid degradation by storing at room temperature in inert atmospheres .
Q. What analytical methods are recommended for quantifying this compound in biological matrices?
High-performance liquid chromatography (HPLC) and liquid chromatography-tandem mass spectrometry (LC-MS/MS) are gold standards. For human plasma, validated methods achieve limits of detection (LOD) as low as 0.219 µg/mL (signal-to-noise ratio ≥3) and limits of quantification (LOQ) of 0.722 µg/mL . Gas chromatography (GC) with cyanosilicone columns (e.g., SP™-2560) is also effective for separating cis/trans isomers in fatty acid methyl ester (FAME) mixtures .
Advanced Research Questions
Q. How can researchers resolve discrepancies in metabolite identification across different analytical platforms?
Discrepancies often arise from variations in ionization efficiency (e.g., ESI vs. APCI) or chromatographic resolution. Cross-validation using orthogonal methods (e.g., NMR for structural confirmation and UPLC-ESI-QTOFMS for high-sensitivity detection) is essential. For example, reduced fenofibric acid taurine was confirmed via NMR after initial MS-based discovery . Robustness testing under varied flow rates and mobile phases (e.g., ±10% organic modifier) further ensures reproducibility .
Q. What strategies optimize stability-indicating methods for this compound under stressed conditions?
Stress testing includes exposure to heat (40–80°C), humidity (75% RH), and oxidative agents (H₂O₂). A validated HPLC method demonstrated robustness with ≤2% deviation in peak area under altered flow rates (0.8–1.2 mL/min) and mobile phase composition (acetonitrile ±5%). Forced degradation studies should monitor hydrolysis products (e.g., fenofibric acid) and glucuronide conjugates .
Q. How do structural modifications to the isopropyl moiety affect this compound’s pharmacokinetics?
X-ray crystallography and molecular docking reveal that rotation of the isopropyl group (torsion angles: −74.73° to 164.60°) influences binding to peroxisome proliferator-activated receptor alpha (PPARα). Comparative studies with clofibrate analogs show that asymmetric conformations reduce metabolic stability but enhance PPARα activation .
Q. What in vitro and in vivo models best predict this compound’s bioavailability and tissue distribution?
In vitro: Caco-2 cell monolayers assess intestinal permeability, while liver microsomes evaluate CYP450-mediated metabolism. In vivo: Perfused pig jejunum models simulate food effects (e.g., lipid-rich diets increase bioavailability via micellar solubilization). This compound’s high lipophilicity (logP ≈5.24) necessitates lipid-based formulations to enhance solubility in preclinical studies .
Q. How can researchers mitigate matrix effects when quantifying this compound in complex biological samples?
Matrix effects are minimized using isotope-labeled internal standards (e.g., 2-chloro fenofibric acid-d6). Solid-phase extraction (SPE) with C18 cartridges effectively removes phospholipids from plasma. Method optimization should include post-column infusion experiments to identify ion suppression zones and adjust gradient elution parameters .
Q. What is the mechanistic basis for this compound’s prodrug activation in different species?
Esterase-mediated hydrolysis converts the methyl ester to fenofibric acid, the active PPARα agonist. Species differences in carboxylesterase isoforms (e.g., higher activity in rodents vs. humans) require cross-validation using recombinant enzyme assays. Pharmacokinetic studies in humans show 60% urinary excretion of fenofibric acid glucuronide, underscoring renal clearance’s role .
Q. How do inter-laboratory variabilities in FAME analysis impact comparative metabolomics studies?
Variabilities arise from column selection (e.g., SP™-2560 for cis/trans resolution vs. Omegawax® for broad-range FAMEs) and derivatization protocols. Harmonization using certified reference materials (CRMs) like the 37-component FAME mix and adherence to AOCS/AOAC guidelines improve cross-study reproducibility .
Eigenschaften
IUPAC Name |
methyl 2-[4-(4-chlorobenzoyl)phenoxy]-2-methylpropanoate | |
---|---|---|
Source | PubChem | |
URL | https://pubchem.ncbi.nlm.nih.gov | |
Description | Data deposited in or computed by PubChem | |
InChI |
InChI=1S/C18H17ClO4/c1-18(2,17(21)22-3)23-15-10-6-13(7-11-15)16(20)12-4-8-14(19)9-5-12/h4-11H,1-3H3 | |
Source | PubChem | |
URL | https://pubchem.ncbi.nlm.nih.gov | |
Description | Data deposited in or computed by PubChem | |
InChI Key |
YNSXVMKCLCCCBA-UHFFFAOYSA-N | |
Source | PubChem | |
URL | https://pubchem.ncbi.nlm.nih.gov | |
Description | Data deposited in or computed by PubChem | |
Canonical SMILES |
CC(C)(C(=O)OC)OC1=CC=C(C=C1)C(=O)C2=CC=C(C=C2)Cl | |
Source | PubChem | |
URL | https://pubchem.ncbi.nlm.nih.gov | |
Description | Data deposited in or computed by PubChem | |
Molecular Formula |
C18H17ClO4 | |
Source | PubChem | |
URL | https://pubchem.ncbi.nlm.nih.gov | |
Description | Data deposited in or computed by PubChem | |
DSSTOX Substance ID |
DTXSID10962165 | |
Record name | Methyl 2-[4-(4-chlorobenzoyl)phenoxy]-2-methylpropanoate | |
Source | EPA DSSTox | |
URL | https://comptox.epa.gov/dashboard/DTXSID10962165 | |
Description | DSSTox provides a high quality public chemistry resource for supporting improved predictive toxicology. | |
Molecular Weight |
332.8 g/mol | |
Source | PubChem | |
URL | https://pubchem.ncbi.nlm.nih.gov | |
Description | Data deposited in or computed by PubChem | |
CAS No. |
42019-07-8 | |
Record name | Propanoic acid, 2-(4-(4-chlorobenzoyl)phenoxy)-2-methyl-, methyl ester | |
Source | ChemIDplus | |
URL | https://pubchem.ncbi.nlm.nih.gov/substance/?source=chemidplus&sourceid=0042019078 | |
Description | ChemIDplus is a free, web search system that provides access to the structure and nomenclature authority files used for the identification of chemical substances cited in National Library of Medicine (NLM) databases, including the TOXNET system. | |
Record name | Methyl 2-[4-(4-chlorobenzoyl)phenoxy]-2-methylpropanoate | |
Source | EPA DSSTox | |
URL | https://comptox.epa.gov/dashboard/DTXSID10962165 | |
Description | DSSTox provides a high quality public chemistry resource for supporting improved predictive toxicology. | |
Retrosynthesis Analysis
AI-Powered Synthesis Planning: Our tool employs the Template_relevance Pistachio, Template_relevance Bkms_metabolic, Template_relevance Pistachio_ringbreaker, Template_relevance Reaxys, Template_relevance Reaxys_biocatalysis model, leveraging a vast database of chemical reactions to predict feasible synthetic routes.
One-Step Synthesis Focus: Specifically designed for one-step synthesis, it provides concise and direct routes for your target compounds, streamlining the synthesis process.
Accurate Predictions: Utilizing the extensive PISTACHIO, BKMS_METABOLIC, PISTACHIO_RINGBREAKER, REAXYS, REAXYS_BIOCATALYSIS database, our tool offers high-accuracy predictions, reflecting the latest in chemical research and data.
Strategy Settings
Precursor scoring | Relevance Heuristic |
---|---|
Min. plausibility | 0.01 |
Model | Template_relevance |
Template Set | Pistachio/Bkms_metabolic/Pistachio_ringbreaker/Reaxys/Reaxys_biocatalysis |
Top-N result to add to graph | 6 |
Feasible Synthetic Routes
Haftungsausschluss und Informationen zu In-Vitro-Forschungsprodukten
Bitte beachten Sie, dass alle Artikel und Produktinformationen, die auf BenchChem präsentiert werden, ausschließlich zu Informationszwecken bestimmt sind. Die auf BenchChem zum Kauf angebotenen Produkte sind speziell für In-vitro-Studien konzipiert, die außerhalb lebender Organismen durchgeführt werden. In-vitro-Studien, abgeleitet von dem lateinischen Begriff "in Glas", beinhalten Experimente, die in kontrollierten Laborumgebungen unter Verwendung von Zellen oder Geweben durchgeführt werden. Es ist wichtig zu beachten, dass diese Produkte nicht als Arzneimittel oder Medikamente eingestuft sind und keine Zulassung der FDA für die Vorbeugung, Behandlung oder Heilung von medizinischen Zuständen, Beschwerden oder Krankheiten erhalten haben. Wir müssen betonen, dass jede Form der körperlichen Einführung dieser Produkte in Menschen oder Tiere gesetzlich strikt untersagt ist. Es ist unerlässlich, sich an diese Richtlinien zu halten, um die Einhaltung rechtlicher und ethischer Standards in Forschung und Experiment zu gewährleisten.