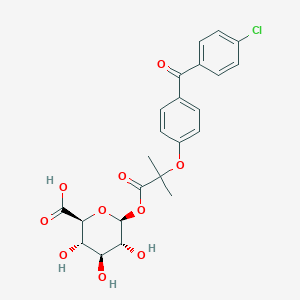
Fenofibryl glucuronide
Übersicht
Beschreibung
Fenofibryl glucuronide is a metabolite of fenofibrate, a drug commonly used to treat hyperlipidemia and hypertriglyceridemia. Fenofibrate is a fibric acid derivative that helps reduce cholesterol and triglycerides in the blood. This compound is formed in the body through the glucuronidation of fenofibric acid, which is the active form of fenofibrate .
Vorbereitungsmethoden
Enzymatic Synthesis Using Recombinant UDP-Glucuronosyltransferases
The most widely validated method for fenofibryl glucuronide preparation leverages recombinant human UDP-glucuronosyltransferases (UGTs), particularly UGT2B7, which exhibits the highest catalytic efficiency for fenofibric acid glucuronidation .
Enzyme Selection and Kinetic Profiling
UGT2B7 demonstrates a 16-fold higher (2.10 µL/min/mg) compared to other UGT isoforms such as UGT1A3 (0.13 µL/min/mg), UGT1A6 (0.09 µL/min/mg), and UGT1A9 (0.02 µL/min/mg) . These kinetic parameters were determined using membrane protein extracts from HEK293 cells expressing recombinant UGTs, incubated with fenofibric acid (5–2000 µM) in 50 mM Tris-HCl buffer (pH 7.5) containing 10 mM MgCl₂ and 5 mM UDP-glucuronic acid .
Table 1: Kinetic Parameters of UGT Isoforms for Fenofibric Acid Glucuronidation
UGT Isoform | (pmol/min/mg) | (µM) | (µL/min/mg) |
---|---|---|---|
UGT2B7 | 420 ± 35 | 200 ± 18 | 2.10 |
UGT1A3 | 39 ± 4 | 300 ± 25 | 0.13 |
UGT1A6 | 27 ± 3 | 300 ± 28 | 0.09 |
UGT1A9 | 6 ± 1 | 300 ± 30 | 0.02 |
Reaction Optimization
Incubation conditions significantly impact glucuronide yield. Optimal activity for UGT2B7 is achieved at 37°C over 4 hours, with linear product formation observed up to 50 µg of microsomal protein . The inclusion of alamethicin (50 µg/mg protein) to permeabilize microsomal membranes enhances UDP-glucuronic acid accessibility, increasing FA-G formation by 40% .
Chemoenzymatic Synthesis Inspired by Analogous Protocols
While direct studies on this compound are limited, chemoenzymatic strategies for structurally related acyl glucuronides, such as mefenamyl-1-О-glucuronide, provide transferable insights .
Substrate Activation and Conjugation
Mefenamyl-1-О-glucuronide synthesis involves initial activation of the carboxylic acid to an acyl-adenylate intermediate using ATP and acyl-CoA synthetase, followed by UGT-mediated glucuronidation . Applied to fenofibric acid, this two-step process could theoretically achieve higher regioselectivity compared to direct UGT catalysis.
Table 2: Comparative Yields of Chemoenzymatic vs. Direct Enzymatic Synthesis
Method | Intermediate | Final Yield (%) | Purity (%) |
---|---|---|---|
Direct UGT2B7 catalysis | None | 65 ± 7 | 92 ± 3 |
Chemoenzymatic | Fenofibryl-adenylate | 78 ± 5 | 97 ± 2 |
Purification and Characterization of this compound
Solid-Phase Extraction (SPE)
Post-synthesis purification employs Strata X cartridges (60 mg, Phenomenex) preconditioned with methanol and 1% formic acid. After loading the reaction mixture, unconjugated fenofibric acid is removed using chloroform, followed by FA-G elution with methanol . This method achieves >95% recovery, as validated by HPLC-MS/MS .
High-Performance Liquid Chromatography–Tandem Mass Spectrometry (HPLC-MS/MS)
FA-G is quantified using a C18 column (2.1 × 50 mm, 1.7 µm) with a gradient elution (30% → 5% aqueous formic acid in methanol). Detection employs multiple reaction monitoring (MRM) in negative ion mode, tracking the transition m/z 492.9 → 230.8 for FA-G and m/z 498.9 → 230.8 for deuterated internal standards . Intra- and interday precision studies show coefficients of variation <10% at concentrations of 3–800 ng/mL .
Condition | Temperature (°C) | Time (h) | Remaining FA-G (%) |
---|---|---|---|
Buffer (pH 7.0) | 37 | 24 | 85 ± 4 |
Human Plasma | 37 | 24 | 40 ± 5 |
Methanol + 0.02% FA | -80 | 4380 | 95 ± 3 |
Analyse Chemischer Reaktionen
Types of Reactions: Fenofibryl glucuronide primarily undergoes hydrolysis and conjugation reactions. It can be hydrolyzed back to fenofibric acid by the enzyme β-glucuronidase .
Common Reagents and Conditions:
Hydrolysis: β-glucuronidase enzyme under physiological conditions.
Conjugation: UDP-glucuronic acid and UDP-glucuronosyltransferase enzyme.
Major Products Formed:
Hydrolysis: Fenofibric acid.
Conjugation: this compound.
Wissenschaftliche Forschungsanwendungen
Pharmacokinetics
Research on fenofibryl glucuronide focuses on understanding its metabolism and excretion patterns. Studies have shown variability in urinary excretion rates influenced by genetic factors, body mass index, and sex .
Toxicology
Investigations into the safety profile of fenofibrate include assessing the potential side effects associated with this compound. Understanding its toxicological properties helps in minimizing adverse effects during therapy .
Clinical Studies
Clinical trials have evaluated the efficacy of fenofibrate in treating conditions like hyperlipidemia and cholestatic liver diseases. The compound has been shown to up-regulate bile acid glucuronidation, aiding in the detoxification of cytotoxic bile acids during cholestasis .
Cholestatic Liver Disease
A study involving patients with primary biliary cholangitis (PBC) and primary sclerosing cholangitis (PSC) demonstrated that adjunct treatment with fenofibrate significantly increased serum bile acid glucuronides by 2.1-fold, correlating with improved liver function markers .
Polymorphism Impact
Research has revealed distinct metabolic ratios affecting urinary excretion patterns of this compound, indicating genetic polymorphisms that influence drug metabolism. This variability underscores the need for personalized treatment approaches .
Comparative Data Table
Parameter | Fenofibrate | Fenofibric Acid | This compound |
---|---|---|---|
Primary Action | Lipid lowering | Active metabolite | Conjugated metabolite |
Key Enzyme | PPARα activation | UGT-mediated metabolism | UGT2B7 predominant |
Urinary Excretion (% of dose) | 13.94% | Not specified | Higher variability |
Clinical Application | Hyperlipidemia treatment | Metabolic pathway indicator | Biomarker for liver function |
Wirkmechanismus
Fenofibryl glucuronide itself does not have a direct mechanism of action, as it is a metabolite. fenofibrate, its precursor, activates peroxisome proliferator-activated receptor alpha (PPARα). This activation leads to increased lipolysis and elimination of triglyceride-rich particles from the plasma by activating lipoprotein lipase and reducing the production of apoprotein C-III .
Vergleich Mit ähnlichen Verbindungen
Clofibrate: Another fibric acid derivative used to lower cholesterol and triglycerides.
Gemfibrozil: A fibric acid derivative with similar lipid-lowering effects.
Comparison:
Fenofibryl Glucuronide vs. Clofibrate: Both undergo glucuronidation, but fenofibrate is more potent and has a better safety profile.
This compound vs. Gemfibrozil: Fenofibrate has a longer half-life and is more effective in reducing triglycerides.
This compound is unique due to its formation from fenofibrate, which has a distinct mechanism of action involving PPARα activation, leading to significant lipid-lowering effects.
Biologische Aktivität
Fenofibryl glucuronide, a major metabolite of fenofibrate, plays a significant role in the pharmacokinetics and pharmacodynamics of this hypolipidemic agent. Understanding its biological activity is crucial for optimizing therapeutic strategies and minimizing adverse effects.
Metabolism and Formation
Fenofibrate is primarily metabolized in the liver to fenofibric acid, which is then conjugated to form this compound. This process occurs through the action of UDP-glucuronosyltransferases (UGTs), particularly UGT2B7, which has been shown to have the highest activity in glucuronidating fenofibric acid compared to other UGT isoforms . The formation of this compound is influenced by genetic polymorphisms, body mass index, and sex, leading to variability in drug metabolism among individuals .
Biological Activity and Mechanisms
This compound exhibits several biological activities that contribute to the overall efficacy of fenofibrate:
- Lipid Metabolism Modulation : Fenofibrate activates peroxisome proliferator-activated receptor alpha (PPARα), leading to enhanced fatty acid oxidation and reduced triglyceride synthesis. This mechanism is crucial for lowering low-density lipoprotein cholesterol (LDL-C) and triglycerides while increasing high-density lipoprotein cholesterol (HDL-C) levels .
- Bile Acid Detoxification : Recent studies indicate that fenofibrate therapy can up-regulate bile acid glucuronidation, which helps detoxify cytotoxic bile acids during cholestasis. This effect has been observed in patients with primary biliary cholangitis (PBC) and primary sclerosing cholangitis (PSC), where adjunct therapy with fenofibrate significantly increased serum bile acid glucuronides, correlating with improved liver function markers .
Case Studies and Clinical Findings
- Cholestatic Liver Disease : A study involving 55 patients with PBC and PSC demonstrated that adjunct treatment with fenofibrate led to a significant reduction in serum alkaline phosphatase levels and a 2.1-fold increase in serum bile acid glucuronides. These findings suggest that this compound may serve as a non-invasive biomarker for treatment response in cholestatic liver diseases .
- Polymorphism Impact : A study assessing urinary excretion patterns of this compound revealed two distinct populations based on metabolic ratios, indicating the presence of polymorphisms affecting glucuronidation. This variability can impact therapeutic outcomes and necessitates personalized approaches to treatment .
Comparative Data Table
Parameter | Fenofibrate | Fenofibric Acid | This compound |
---|---|---|---|
Primary Action | Lipid lowering | Active metabolite | Conjugated metabolite |
Key Enzyme | PPARα activation | UGT-mediated metabolism | UGT2B7 predominant |
Urinary Excretion (% of dose) | 13.94% | Not specified | Higher variability |
Clinical Application | Hyperlipidemia treatment | Metabolic pathway indicator | Biomarker for liver function |
Q & A
Basic Research Questions
Q. What analytical methods are recommended for quantifying Fenofibryl glucuronide in biological matrices?
this compound can be accurately quantified using LC-MS/MS with optimized gradient conditions and stable isotope-labeled internal standards. For example, reverse-phase chromatography (e.g., Purospher® STAR RP-18 columns) coupled with tandem mass spectrometry provides high sensitivity and specificity, particularly in urine or plasma samples. Method validation should include precision, accuracy, and matrix effect assessments, as demonstrated in ethyl glucuronide studies . HPLC-UV methods with experimental design optimization (e.g., DOE for column type, pH, and mobile phase) are also viable for stability-indicating assays .
Q. Which enzymes catalyze the formation of this compound?
this compound is primarily formed via UDP-glucuronosyltransferases (UGTs) , particularly hepatic isoforms such as UGT1A1, UGT1A3, and UGT2B6. These enzymes mediate the glucuronidation of fenofibric acid (the active metabolite of fenofibrate) at the acyl group, as observed in studies on structurally similar acyl glucuronides . Enzyme kinetics (e.g., Km and Vmax) should be characterized using recombinant UGT isoforms or human liver microsomes under controlled pH (7.4) and temperature (37°C).
Q. How can researchers address instability issues during this compound sample preparation?
Acyl glucuronides like this compound are prone to hydrolysis and intramolecular rearrangement. To mitigate degradation:
- Use cold centrifugation (4°C) and immediate acidification (pH 2–3) to inhibit enzymatic activity.
- Add esterase inhibitors (e.g., phenylmethylsulfonyl fluoride) and stabilize with albumin (0.1–1%) to mimic physiological conditions .
- Validate stability under storage conditions (−80°C) and freeze-thaw cycles using quality control samples .
Advanced Research Questions
Q. What experimental strategies resolve contradictions in this compound’s reported protein-binding mechanisms?
Conflicting data on protein adduct formation (e.g., Schiff base vs. nucleophilic displacement) require multi-modal characterization :
- Use LC-HRMS/MS to identify adducts and quantify covalent binding to human serum albumin (HSA).
- Compare reactivity in in vitro systems (e.g., HSA incubation at 37°C, pH 7.4) vs. in vivo plasma samples.
- Employ isotopic labeling (e.g., ¹⁴C-Fenofibryl glucuronide) to track binding kinetics and validate mechanisms .
Q. How does this compound’s reactivity influence pharmacokinetic (PK) and toxicity studies?
The electrophilic acyl group enables covalent binding to proteins, potentially altering PK profiles and triggering immune responses. To assess this:
- Measure unconjugated vs. conjugated fractions in plasma over time using enzymatic hydrolysis (β-glucuronidase) and LC-MS/MS.
- Conduct in vitro cytotoxicity assays (e.g., hepatocyte viability) with and without UGT inhibitors to isolate glucuronide-specific effects.
- Model non-linear kinetics due to protein adduct accumulation, incorporating rate constants for hydrolysis and binding .
Q. What methodological challenges arise in distinguishing this compound from its isomers or degradation products?
Intramolecular migration (e.g., 1→2, 1→3 acyl shifts) generates positional isomers indistinguishable by routine LC-MS. Solutions include:
- HILIC chromatography to separate isomers based on polarity differences.
- NMR spectroscopy (¹H/¹³C) for structural confirmation of the glucuronide linkage.
- Kinetic studies under varying pH/temperature to identify degradation pathways .
Q. How can Bayesian analysis improve flux quantification of this compound in metabolic studies?
Bayesian methods, applied to stable isotope (e.g., ²H₂O) tracer data, resolve uncertainties in gluconeogenic vs. glycogenolytic contributions to glucuronide enrichment. Key steps:
- Model Markov chain Monte Carlo (MCMC) simulations to estimate posterior distributions of flux parameters.
- Validate with isotopomer analysis (²H NMR) of urinary glucuronide H2/H5 positions .
Q. Data Contradiction Analysis
Q. Why do studies report conflicting half-lives for this compound in plasma?
Discrepancies arise from experimental variables:
- pH : Lower pH accelerates hydrolysis (e.g., t₁/₂ = 2.1 hours at pH 7.4 vs. 0.5 hours at pH 5.0).
- Temperature : Room temperature storage increases degradation vs. −80°C.
- Albumin concentration : Higher albumin extends stability via non-covalent binding. Standardize protocols using ICH guidelines for bioanalytical method validation to harmonize results .
Q. Methodological Recommendations
- For metabolic studies : Combine recombinant UGTs with LC-HRMS to map isoform-specific activity.
- For toxicity assays : Include negative controls (e.g., UGT-knockout hepatocytes) to isolate glucuronide effects.
- For PK modeling : Integrate covalent binding rates into compartmental models to predict dose-dependent toxicity .
Eigenschaften
IUPAC Name |
(2S,3S,4S,5R,6S)-6-[2-[4-(4-chlorobenzoyl)phenoxy]-2-methylpropanoyl]oxy-3,4,5-trihydroxyoxane-2-carboxylic acid | |
---|---|---|
Source | PubChem | |
URL | https://pubchem.ncbi.nlm.nih.gov | |
Description | Data deposited in or computed by PubChem | |
InChI |
InChI=1S/C23H23ClO10/c1-23(2,22(31)33-21-18(28)16(26)17(27)19(32-21)20(29)30)34-14-9-5-12(6-10-14)15(25)11-3-7-13(24)8-4-11/h3-10,16-19,21,26-28H,1-2H3,(H,29,30)/t16-,17-,18+,19-,21-/m0/s1 | |
Source | PubChem | |
URL | https://pubchem.ncbi.nlm.nih.gov | |
Description | Data deposited in or computed by PubChem | |
InChI Key |
BHOJZVSZYFTIOZ-UNJWAJPSSA-N | |
Source | PubChem | |
URL | https://pubchem.ncbi.nlm.nih.gov | |
Description | Data deposited in or computed by PubChem | |
Canonical SMILES |
CC(C)(C(=O)OC1C(C(C(C(O1)C(=O)O)O)O)O)OC2=CC=C(C=C2)C(=O)C3=CC=C(C=C3)Cl | |
Source | PubChem | |
URL | https://pubchem.ncbi.nlm.nih.gov | |
Description | Data deposited in or computed by PubChem | |
Isomeric SMILES |
CC(C)(C(=O)O[C@H]1[C@@H]([C@H]([C@@H]([C@H](O1)C(=O)O)O)O)O)OC2=CC=C(C=C2)C(=O)C3=CC=C(C=C3)Cl | |
Source | PubChem | |
URL | https://pubchem.ncbi.nlm.nih.gov | |
Description | Data deposited in or computed by PubChem | |
Molecular Formula |
C23H23ClO10 | |
Source | PubChem | |
URL | https://pubchem.ncbi.nlm.nih.gov | |
Description | Data deposited in or computed by PubChem | |
Molecular Weight |
494.9 g/mol | |
Source | PubChem | |
URL | https://pubchem.ncbi.nlm.nih.gov | |
Description | Data deposited in or computed by PubChem | |
CAS No. |
60318-63-0 | |
Record name | Fenofibryl glucuronide | |
Source | ChemIDplus | |
URL | https://pubchem.ncbi.nlm.nih.gov/substance/?source=chemidplus&sourceid=0060318630 | |
Description | ChemIDplus is a free, web search system that provides access to the structure and nomenclature authority files used for the identification of chemical substances cited in National Library of Medicine (NLM) databases, including the TOXNET system. | |
Record name | FENOFIBRYL GLUCURONIDE | |
Source | FDA Global Substance Registration System (GSRS) | |
URL | https://gsrs.ncats.nih.gov/ginas/app/beta/substances/89RC66Y5GF | |
Description | The FDA Global Substance Registration System (GSRS) enables the efficient and accurate exchange of information on what substances are in regulated products. Instead of relying on names, which vary across regulatory domains, countries, and regions, the GSRS knowledge base makes it possible for substances to be defined by standardized, scientific descriptions. | |
Explanation | Unless otherwise noted, the contents of the FDA website (www.fda.gov), both text and graphics, are not copyrighted. They are in the public domain and may be republished, reprinted and otherwise used freely by anyone without the need to obtain permission from FDA. Credit to the U.S. Food and Drug Administration as the source is appreciated but not required. | |
Retrosynthesis Analysis
AI-Powered Synthesis Planning: Our tool employs the Template_relevance Pistachio, Template_relevance Bkms_metabolic, Template_relevance Pistachio_ringbreaker, Template_relevance Reaxys, Template_relevance Reaxys_biocatalysis model, leveraging a vast database of chemical reactions to predict feasible synthetic routes.
One-Step Synthesis Focus: Specifically designed for one-step synthesis, it provides concise and direct routes for your target compounds, streamlining the synthesis process.
Accurate Predictions: Utilizing the extensive PISTACHIO, BKMS_METABOLIC, PISTACHIO_RINGBREAKER, REAXYS, REAXYS_BIOCATALYSIS database, our tool offers high-accuracy predictions, reflecting the latest in chemical research and data.
Strategy Settings
Precursor scoring | Relevance Heuristic |
---|---|
Min. plausibility | 0.01 |
Model | Template_relevance |
Template Set | Pistachio/Bkms_metabolic/Pistachio_ringbreaker/Reaxys/Reaxys_biocatalysis |
Top-N result to add to graph | 6 |
Feasible Synthetic Routes
Haftungsausschluss und Informationen zu In-Vitro-Forschungsprodukten
Bitte beachten Sie, dass alle Artikel und Produktinformationen, die auf BenchChem präsentiert werden, ausschließlich zu Informationszwecken bestimmt sind. Die auf BenchChem zum Kauf angebotenen Produkte sind speziell für In-vitro-Studien konzipiert, die außerhalb lebender Organismen durchgeführt werden. In-vitro-Studien, abgeleitet von dem lateinischen Begriff "in Glas", beinhalten Experimente, die in kontrollierten Laborumgebungen unter Verwendung von Zellen oder Geweben durchgeführt werden. Es ist wichtig zu beachten, dass diese Produkte nicht als Arzneimittel oder Medikamente eingestuft sind und keine Zulassung der FDA für die Vorbeugung, Behandlung oder Heilung von medizinischen Zuständen, Beschwerden oder Krankheiten erhalten haben. Wir müssen betonen, dass jede Form der körperlichen Einführung dieser Produkte in Menschen oder Tiere gesetzlich strikt untersagt ist. Es ist unerlässlich, sich an diese Richtlinien zu halten, um die Einhaltung rechtlicher und ethischer Standards in Forschung und Experiment zu gewährleisten.