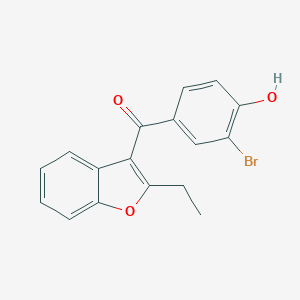
Bromobenzarone
Übersicht
Beschreibung
Bromobenzarone is a metabolite of benzbromarone, a benzofuran derivative known for its uricosuric properties. It is primarily used in the treatment of gout and hyperuricemia by lowering serum urate levels and increasing urinary urate excretion .
Wissenschaftliche Forschungsanwendungen
Bromobenzarone has several scientific research applications, including:
Chemistry: Used as an intermediate in the synthesis of various organic compounds.
Biology: Studied for its effects on cellular processes and metabolic pathways.
Medicine: Investigated for its potential therapeutic effects in treating hyperuricemia and gout.
Industry: Utilized in the production of pharmaceuticals and other chemical products.
Wirkmechanismus
Target of Action
Bromobenzarone primarily targets the uric acid in the body . It is a uricosuric agent, meaning it increases the excretion of uric acid in the urine .
Mode of Action
This compound interacts with its targets by decreasing the reabsorption of uric acid . This interaction results in a reduction of serum uric acid levels and an increase in urinary uric acid excretion .
Biochemical Pathways
This compound affects the biochemical pathways related to purine metabolism . It has an effect on enzymes involved in purine metabolism, which contributes to its urate-lowering effect .
Pharmacokinetics
This compound’s pharmacokinetics involve absorption, distribution, metabolism, and excretion (ADME). About 50% of a single, non-micronised dose is absorbed and dehalogenated in the liver to form this compound and benzarone . Excretion occurs primarily via the bile and faeces and to a lesser extent in the urine . Hydroxylation has been shown to be the primary metabolic pathway .
Result of Action
The result of this compound’s action is a significant reduction in serum uric acid levels and an increase in urinary uric acid excretion . This makes it effective in the treatment of conditions like gout and hyperuricemia .
Action Environment
The action, efficacy, and stability of this compound can be influenced by environmental factors. For instance, food intake can retard drug absorption . Additionally, concomitant administration of certain drugs like pyrazinamide or aspirin can decrease the effectiveness of this compound .
Biochemische Analyse
Biochemical Properties
Bromobenzarone has been shown to interact with various enzymes and proteins. It is metabolized predominantly by cytochrome P450 (CYP) 2C9 . The nature of these interactions involves the primary metabolic pathway of hydroxylation .
Cellular Effects
It is known that this compound can influence cell function through its interactions with enzymes like CYP2C9 .
Molecular Mechanism
This compound exerts its effects at the molecular level through various mechanisms. One key mechanism is through the inhibition of CYP2C9, which plays a critical role in its metabolism . This interaction can lead to changes in gene expression and enzyme activity.
Temporal Effects in Laboratory Settings
It is known that this compound can be metabolized through hydroxylation, suggesting that it may have stability and degradation properties related to this process .
Metabolic Pathways
This compound is involved in metabolic pathways related to the cytochrome P450 system, specifically CYP2C9 . It undergoes hydroxylation, which is a primary metabolic pathway .
Vorbereitungsmethoden
Synthetic Routes and Reaction Conditions: Bromobenzarone is synthesized through the debromination of benzbromarone. The primary metabolic pathway involves hydroxylation rather than debromination . The synthesis typically involves the use of bromine and benzofuran derivatives under controlled conditions to achieve the desired product.
Industrial Production Methods: Industrial production of this compound follows similar synthetic routes but on a larger scale. The process involves stringent quality control measures to ensure the purity and efficacy of the compound. The use of advanced techniques such as high-performance liquid chromatography (HPLC) is common to monitor the synthesis process .
Analyse Chemischer Reaktionen
Types of Reactions: Bromobenzarone undergoes various chemical reactions, including:
Oxidation: This reaction involves the addition of oxygen or the removal of hydrogen, leading to the formation of hydroxylated metabolites.
Reduction: This reaction involves the addition of hydrogen or the removal of oxygen, although it is less common for this compound.
Substitution: This reaction involves the replacement of a functional group with another, such as the substitution of bromine atoms.
Common Reagents and Conditions:
Oxidizing Agents: Hydrogen peroxide, potassium permanganate.
Reducing Agents: Sodium borohydride, lithium aluminum hydride.
Substitution Reagents: Halogenating agents like bromine, chlorine.
Major Products Formed: The major products formed from these reactions include hydroxylated derivatives and other substituted compounds, which are often used in further chemical synthesis and research .
Vergleich Mit ähnlichen Verbindungen
Benzbromarone: The parent compound, known for its uricosuric properties.
Probenecid: Another uricosuric agent used in the treatment of gout.
Sulfinpyrazone: A uricosuric drug with similar effects.
Uniqueness: Bromobenzarone is unique due to its specific metabolic pathway and the formation of hydroxylated metabolites. Unlike other uricosuric agents, it can be administered in a once-daily regimen, making it more convenient for patients .
Eigenschaften
IUPAC Name |
(3-bromo-4-hydroxyphenyl)-(2-ethyl-1-benzofuran-3-yl)methanone | |
---|---|---|
Source | PubChem | |
URL | https://pubchem.ncbi.nlm.nih.gov | |
Description | Data deposited in or computed by PubChem | |
InChI |
InChI=1S/C17H13BrO3/c1-2-14-16(11-5-3-4-6-15(11)21-14)17(20)10-7-8-13(19)12(18)9-10/h3-9,19H,2H2,1H3 | |
Source | PubChem | |
URL | https://pubchem.ncbi.nlm.nih.gov | |
Description | Data deposited in or computed by PubChem | |
InChI Key |
QXZXBNDIHSUZDF-UHFFFAOYSA-N | |
Source | PubChem | |
URL | https://pubchem.ncbi.nlm.nih.gov | |
Description | Data deposited in or computed by PubChem | |
Canonical SMILES |
CCC1=C(C2=CC=CC=C2O1)C(=O)C3=CC(=C(C=C3)O)Br | |
Source | PubChem | |
URL | https://pubchem.ncbi.nlm.nih.gov | |
Description | Data deposited in or computed by PubChem | |
Molecular Formula |
C17H13BrO3 | |
Source | PubChem | |
URL | https://pubchem.ncbi.nlm.nih.gov | |
Description | Data deposited in or computed by PubChem | |
DSSTOX Substance ID |
DTXSID80915345 | |
Record name | (3-Bromo-4-hydroxyphenyl)(2-ethyl-1-benzofuran-3-yl)methanone | |
Source | EPA DSSTox | |
URL | https://comptox.epa.gov/dashboard/DTXSID80915345 | |
Description | DSSTox provides a high quality public chemistry resource for supporting improved predictive toxicology. | |
Molecular Weight |
345.2 g/mol | |
Source | PubChem | |
URL | https://pubchem.ncbi.nlm.nih.gov | |
Description | Data deposited in or computed by PubChem | |
CAS No. |
94729-09-6 | |
Record name | Bromobenzarone | |
Source | ChemIDplus | |
URL | https://pubchem.ncbi.nlm.nih.gov/substance/?source=chemidplus&sourceid=0094729096 | |
Description | ChemIDplus is a free, web search system that provides access to the structure and nomenclature authority files used for the identification of chemical substances cited in National Library of Medicine (NLM) databases, including the TOXNET system. | |
Record name | (3-Bromo-4-hydroxyphenyl)(2-ethyl-1-benzofuran-3-yl)methanone | |
Source | EPA DSSTox | |
URL | https://comptox.epa.gov/dashboard/DTXSID80915345 | |
Description | DSSTox provides a high quality public chemistry resource for supporting improved predictive toxicology. | |
Retrosynthesis Analysis
AI-Powered Synthesis Planning: Our tool employs the Template_relevance Pistachio, Template_relevance Bkms_metabolic, Template_relevance Pistachio_ringbreaker, Template_relevance Reaxys, Template_relevance Reaxys_biocatalysis model, leveraging a vast database of chemical reactions to predict feasible synthetic routes.
One-Step Synthesis Focus: Specifically designed for one-step synthesis, it provides concise and direct routes for your target compounds, streamlining the synthesis process.
Accurate Predictions: Utilizing the extensive PISTACHIO, BKMS_METABOLIC, PISTACHIO_RINGBREAKER, REAXYS, REAXYS_BIOCATALYSIS database, our tool offers high-accuracy predictions, reflecting the latest in chemical research and data.
Strategy Settings
Precursor scoring | Relevance Heuristic |
---|---|
Min. plausibility | 0.01 |
Model | Template_relevance |
Template Set | Pistachio/Bkms_metabolic/Pistachio_ringbreaker/Reaxys/Reaxys_biocatalysis |
Top-N result to add to graph | 6 |
Feasible Synthetic Routes
Q1: How is benzbromarone metabolized in the human body?
A1: Benzbromarone undergoes hepatic metabolism, primarily resulting in two hydroxylated metabolites: 1'-hydroxybenzbromarone (M1) and 6-hydroxybenzbromarone (M2). [, ] These metabolites are formed through hydroxylation reactions. [] Contrary to previous assumptions, debrominated metabolites like bromobenzarone and benzarone are not detected in significant amounts. [, ]
Q2: How are benzbromarone and its metabolites excreted?
A2: Urinary excretion of benzbromarone and its metabolites is minimal. [] Research shows that biliary excretion plays a significant role in the elimination of benzbromarone, M1, and M2. [] This suggests a potential enterohepatic recirculation, influencing the drug's pharmacokinetics. []
Q3: Are there any known genetic polymorphisms affecting benzbromarone elimination?
A3: Yes, research suggests a genetically determined polymorphism influencing benzbromarone elimination from plasma, potentially inherited in an autosomal recessive manner. [] This highlights the importance of understanding individual variations in drug metabolism.
Q4: What analytical techniques are commonly used to study benzbromarone and its metabolites?
A4: Several analytical methods are employed to analyze benzbromarone and its metabolites. These include high-performance liquid chromatography (HPLC), gas chromatography-mass spectrometry (GC-MS), and gas chromatography with electron capture detection (GC-ECD). [, , ] These techniques allow for the identification and quantification of these compounds in various biological matrices.
Haftungsausschluss und Informationen zu In-Vitro-Forschungsprodukten
Bitte beachten Sie, dass alle Artikel und Produktinformationen, die auf BenchChem präsentiert werden, ausschließlich zu Informationszwecken bestimmt sind. Die auf BenchChem zum Kauf angebotenen Produkte sind speziell für In-vitro-Studien konzipiert, die außerhalb lebender Organismen durchgeführt werden. In-vitro-Studien, abgeleitet von dem lateinischen Begriff "in Glas", beinhalten Experimente, die in kontrollierten Laborumgebungen unter Verwendung von Zellen oder Geweben durchgeführt werden. Es ist wichtig zu beachten, dass diese Produkte nicht als Arzneimittel oder Medikamente eingestuft sind und keine Zulassung der FDA für die Vorbeugung, Behandlung oder Heilung von medizinischen Zuständen, Beschwerden oder Krankheiten erhalten haben. Wir müssen betonen, dass jede Form der körperlichen Einführung dieser Produkte in Menschen oder Tiere gesetzlich strikt untersagt ist. Es ist unerlässlich, sich an diese Richtlinien zu halten, um die Einhaltung rechtlicher und ethischer Standards in Forschung und Experiment zu gewährleisten.