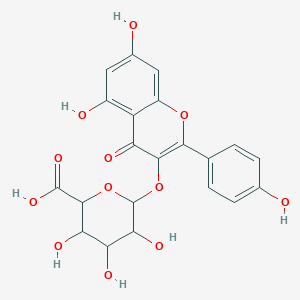
Kaempferol 3-glucuronide
Übersicht
Beschreibung
Kaempferol-3-glucuronide is a flavonol glycoside, specifically a conjugated metabolite of kaempferol. It is known for its significant anti-inflammatory properties and is found naturally in various plants, including Fragaria species . This compound has garnered attention due to its potential therapeutic applications and its role in various biological processes.
Wissenschaftliche Forschungsanwendungen
Kaempferol-3-glucuronid hat ein breites Anwendungsspektrum in der wissenschaftlichen Forschung:
Chemie: Es wird als Referenzverbindung in analytischen Studien und als Vorläufer bei der Synthese anderer Flavonoidderivate verwendet.
Biologie: Die Verbindung wird hinsichtlich ihrer Rolle im Pflanzenstoffwechsel und ihrer Auswirkungen auf zelluläre Prozesse untersucht.
Industrie: Es wird aufgrund seiner gesundheitlichen Vorteile in der Formulierung von Nahrungsergänzungsmitteln und funktionellen Lebensmitteln verwendet.
5. Wirkmechanismus
Kaempferol-3-glucuronid übt seine Wirkungen über verschiedene molekulare Zielstrukturen und Pfade aus:
Entzündungshemmende Wirkung: Es hemmt proinflammatorische Mediatoren wie Interleukin-1β, Stickstoffmonoxid, Prostaglandin E2 und Leukotrien B4. Es erhöht auch die Sekretion des entzündungshemmenden Zytokins Interleukin-10.
Antioxidative Aktivität: Die Verbindung fängt reaktive Sauerstoffspezies ab und verstärkt die Aktivität antioxidativer Enzyme.
Krebshemmende Wirkungen: Es induziert Apoptose in Krebszellen und hemmt die Zellproliferation über Pfade, die Akt und andere Signalmoleküle betreffen.
Wirkmechanismus
Target of Action
Kaempferol 3-glucuronide, a glucuronide metabolite of the flavonoid kaempferol, has been shown to directly target the AKT PH domain . The AKT pathway plays a crucial role in multiple cellular processes such as cell growth, proliferation, and survival .
Mode of Action
this compound interacts with its targets by activating the AKT/GSK3β signaling pathway . This activation leads to improved glucose metabolism .
Biochemical Pathways
The primary biochemical pathway affected by this compound is the AKT/GSK3β signaling pathway . The activation of this pathway can lead to various downstream effects, including the regulation of cell growth and survival, as well as improved glucose metabolism .
Pharmacokinetics
The pharmacokinetics of this compound involve its absorption, distribution, metabolism, and excretion (ADME). Earlier studies have demonstrated that the 3-glucuronide conjugate of kaempferol is the main component detected in plasma and urine . The urine excretion of consumed kaempferol was approximately 1.9% to 2.5% .
Result of Action
The activation of the AKT/GSK3β signaling pathway by this compound leads to improved glucose metabolism . This can result in various molecular and cellular effects, including the regulation of cell growth and survival .
Action Environment
The action of this compound can be influenced by various environmental factors. For instance, the bioavailability and efficacy of this compound can be enhanced through the development of nanoparticles as regulated drug delivery systems . This approach can help overcome the challenges associated with its poor water solubility, poor permeability, and poor bioavailability .
Safety and Hazards
Biochemische Analyse
Biochemical Properties
Kaempferol 3-glucuronide interacts with various biomolecules, contributing to its biochemical properties. It has been shown to significantly inhibit pro-inflammatory mediators such as IL-1β, NO, PGE2, and LTB4 . It also upregulates the secretion of the anti-inflammatory cytokine IL-10 .
Cellular Effects
This compound has been shown to have significant effects on various types of cells and cellular processes. For instance, it has been found to induce Akt activity in mouse liver homogenates and increase glucose consumption in HepG2 cells . It also has anti-inflammatory effects in LPS-induced RAW 264.7 cells and mice models .
Molecular Mechanism
The molecular mechanism of this compound involves its interaction with various biomolecules. It has been shown to target the AKT PH domain and activate the AKT/GSK3β signaling pathway, thereby improving glucose metabolism .
Temporal Effects in Laboratory Settings
It has been shown to reduce inflammation in a dose-dependent manner both in vitro and in vivo .
Dosage Effects in Animal Models
In animal models, this compound has been shown to reduce inflammation in a dose-dependent manner
Metabolic Pathways
It has been shown to influence the AKT/GSK3β signaling pathway, which plays a crucial role in cellular metabolism .
Transport and Distribution
It has been suggested that it is predominantly absorbed in the small intestine via active transport, passive diffusion, and facilitated diffusion .
Subcellular Localization
It has been suggested that it is predominantly absorbed in the small intestine
Vorbereitungsmethoden
Synthetic Routes and Reaction Conditions: The synthesis of kaempferol-3-glucuronide typically involves the glucuronidation of kaempferol. This process can be achieved through enzymatic or chemical methods. Enzymatic glucuronidation uses UDP-glucuronosyltransferases (UGTs) to transfer glucuronic acid to kaempferol. Chemical synthesis often involves the use of glucuronic acid derivatives under acidic or basic conditions to facilitate the conjugation.
Industrial Production Methods: Industrial production of kaempferol-3-glucuronide may involve biotechnological approaches, such as microbial fermentation, where genetically engineered microorganisms express UGTs to produce the compound. Alternatively, chemical synthesis on a large scale can be optimized for higher yields and purity, using advanced purification techniques like chromatography.
Analyse Chemischer Reaktionen
Arten von Reaktionen: Kaempferol-3-glucuronid unterliegt verschiedenen chemischen Reaktionen, darunter:
Oxidation: Diese Reaktion kann zur Bildung von Chinonen oder anderen oxidierten Derivaten führen.
Reduktion: Reduktionsreaktionen können die Verbindung in ihre entsprechenden Alkohole oder andere reduzierte Formen umwandeln.
Substitution: Nucleophile Substitutionsreaktionen können die Glucuronsäureeinheit durch andere funktionelle Gruppen ersetzen.
Häufige Reagenzien und Bedingungen:
Oxidation: Häufige Oxidationsmittel sind Wasserstoffperoxid und Kaliumpermanganat.
Reduktion: Reduktionsmittel wie Natriumborhydrid oder Lithiumaluminiumhydrid werden häufig verwendet.
Substitution: Bedingungen können Nucleophile wie Thiole oder Amine unter basischem oder neutralem pH-Wert umfassen.
Hauptprodukte: Die aus diesen Reaktionen entstehenden Hauptprodukte hängen von den spezifischen Bedingungen und verwendeten Reagenzien ab. Beispielsweise kann Oxidation Kaempferolchinone liefern, während Reduktion Kaempferolalkohole erzeugen kann.
Vergleich Mit ähnlichen Verbindungen
Kaempferol-3-glucuronid kann mit anderen Flavonolglykosiden wie Quercetin-3-glucuronid und Myricetin-3-glucuronid verglichen werden:
Quercetin-3-glucuronid: Ähnlich in der Struktur, unterscheidet sich jedoch im Hydroxylierungsmuster auf dem Flavonoidgerüst. Es zeigt auch entzündungshemmende und antioxidative Eigenschaften.
Myricetin-3-glucuronid: Enthält zusätzliche Hydroxylgruppen, die seine antioxidative Kapazität erhöhen, aber auch seine Löslichkeit und Bioverfügbarkeit beeinflussen können.
Kaempferol-3-glucuronid ist aufgrund seines spezifischen Glucuronidierungsmusters einzigartig, das seine biologische Aktivität und sein therapeutisches Potenzial beeinflusst.
Eigenschaften
IUPAC Name |
6-[5,7-dihydroxy-2-(4-hydroxyphenyl)-4-oxochromen-3-yl]oxy-3,4,5-trihydroxyoxane-2-carboxylic acid | |
---|---|---|
Details | Computed by LexiChem 2.6.6 (PubChem release 2019.06.18) | |
Source | PubChem | |
URL | https://pubchem.ncbi.nlm.nih.gov | |
Description | Data deposited in or computed by PubChem | |
InChI |
InChI=1S/C21H18O12/c22-8-3-1-7(2-4-8)17-18(13(25)12-10(24)5-9(23)6-11(12)31-17)32-21-16(28)14(26)15(27)19(33-21)20(29)30/h1-6,14-16,19,21-24,26-28H,(H,29,30) | |
Details | Computed by InChI 1.0.5 (PubChem release 2019.06.18) | |
Source | PubChem | |
URL | https://pubchem.ncbi.nlm.nih.gov | |
Description | Data deposited in or computed by PubChem | |
InChI Key |
FNTJVYCFNVUBOL-UHFFFAOYSA-N | |
Details | Computed by InChI 1.0.5 (PubChem release 2019.06.18) | |
Source | PubChem | |
URL | https://pubchem.ncbi.nlm.nih.gov | |
Description | Data deposited in or computed by PubChem | |
Canonical SMILES |
C1=CC(=CC=C1C2=C(C(=O)C3=C(C=C(C=C3O2)O)O)OC4C(C(C(C(O4)C(=O)O)O)O)O)O | |
Details | Computed by OEChem 2.1.5 (PubChem release 2019.06.18) | |
Source | PubChem | |
URL | https://pubchem.ncbi.nlm.nih.gov | |
Description | Data deposited in or computed by PubChem | |
Molecular Formula |
C21H18O12 | |
Details | Computed by PubChem 2.1 (PubChem release 2019.06.18) | |
Source | PubChem | |
URL | https://pubchem.ncbi.nlm.nih.gov | |
Description | Data deposited in or computed by PubChem | |
Molecular Weight |
462.4 g/mol | |
Details | Computed by PubChem 2.1 (PubChem release 2021.05.07) | |
Source | PubChem | |
URL | https://pubchem.ncbi.nlm.nih.gov | |
Description | Data deposited in or computed by PubChem | |
Physical Description |
Solid | |
Record name | Kaempferol 3-glucuronide | |
Source | Human Metabolome Database (HMDB) | |
URL | http://www.hmdb.ca/metabolites/HMDB0029500 | |
Description | The Human Metabolome Database (HMDB) is a freely available electronic database containing detailed information about small molecule metabolites found in the human body. | |
Explanation | HMDB is offered to the public as a freely available resource. Use and re-distribution of the data, in whole or in part, for commercial purposes requires explicit permission of the authors and explicit acknowledgment of the source material (HMDB) and the original publication (see the HMDB citing page). We ask that users who download significant portions of the database cite the HMDB paper in any resulting publications. | |
CAS No. |
22688-78-4 | |
Record name | Kaempferol 3-O-β-D-glucuronide | |
Source | European Chemicals Agency (ECHA) | |
URL | https://echa.europa.eu/information-on-chemicals | |
Description | The European Chemicals Agency (ECHA) is an agency of the European Union which is the driving force among regulatory authorities in implementing the EU's groundbreaking chemicals legislation for the benefit of human health and the environment as well as for innovation and competitiveness. | |
Explanation | Use of the information, documents and data from the ECHA website is subject to the terms and conditions of this Legal Notice, and subject to other binding limitations provided for under applicable law, the information, documents and data made available on the ECHA website may be reproduced, distributed and/or used, totally or in part, for non-commercial purposes provided that ECHA is acknowledged as the source: "Source: European Chemicals Agency, http://echa.europa.eu/". Such acknowledgement must be included in each copy of the material. ECHA permits and encourages organisations and individuals to create links to the ECHA website under the following cumulative conditions: Links can only be made to webpages that provide a link to the Legal Notice page. | |
Record name | Kaempferol 3-glucuronide | |
Source | Human Metabolome Database (HMDB) | |
URL | http://www.hmdb.ca/metabolites/HMDB0029500 | |
Description | The Human Metabolome Database (HMDB) is a freely available electronic database containing detailed information about small molecule metabolites found in the human body. | |
Explanation | HMDB is offered to the public as a freely available resource. Use and re-distribution of the data, in whole or in part, for commercial purposes requires explicit permission of the authors and explicit acknowledgment of the source material (HMDB) and the original publication (see the HMDB citing page). We ask that users who download significant portions of the database cite the HMDB paper in any resulting publications. | |
Melting Point |
189 - 190.5 °C | |
Record name | Kaempferol 3-glucuronide | |
Source | Human Metabolome Database (HMDB) | |
URL | http://www.hmdb.ca/metabolites/HMDB0029500 | |
Description | The Human Metabolome Database (HMDB) is a freely available electronic database containing detailed information about small molecule metabolites found in the human body. | |
Explanation | HMDB is offered to the public as a freely available resource. Use and re-distribution of the data, in whole or in part, for commercial purposes requires explicit permission of the authors and explicit acknowledgment of the source material (HMDB) and the original publication (see the HMDB citing page). We ask that users who download significant portions of the database cite the HMDB paper in any resulting publications. | |
Retrosynthesis Analysis
AI-Powered Synthesis Planning: Our tool employs the Template_relevance Pistachio, Template_relevance Bkms_metabolic, Template_relevance Pistachio_ringbreaker, Template_relevance Reaxys, Template_relevance Reaxys_biocatalysis model, leveraging a vast database of chemical reactions to predict feasible synthetic routes.
One-Step Synthesis Focus: Specifically designed for one-step synthesis, it provides concise and direct routes for your target compounds, streamlining the synthesis process.
Accurate Predictions: Utilizing the extensive PISTACHIO, BKMS_METABOLIC, PISTACHIO_RINGBREAKER, REAXYS, REAXYS_BIOCATALYSIS database, our tool offers high-accuracy predictions, reflecting the latest in chemical research and data.
Strategy Settings
Precursor scoring | Relevance Heuristic |
---|---|
Min. plausibility | 0.01 |
Model | Template_relevance |
Template Set | Pistachio/Bkms_metabolic/Pistachio_ringbreaker/Reaxys/Reaxys_biocatalysis |
Top-N result to add to graph | 6 |
Feasible Synthetic Routes
Haftungsausschluss und Informationen zu In-Vitro-Forschungsprodukten
Bitte beachten Sie, dass alle Artikel und Produktinformationen, die auf BenchChem präsentiert werden, ausschließlich zu Informationszwecken bestimmt sind. Die auf BenchChem zum Kauf angebotenen Produkte sind speziell für In-vitro-Studien konzipiert, die außerhalb lebender Organismen durchgeführt werden. In-vitro-Studien, abgeleitet von dem lateinischen Begriff "in Glas", beinhalten Experimente, die in kontrollierten Laborumgebungen unter Verwendung von Zellen oder Geweben durchgeführt werden. Es ist wichtig zu beachten, dass diese Produkte nicht als Arzneimittel oder Medikamente eingestuft sind und keine Zulassung der FDA für die Vorbeugung, Behandlung oder Heilung von medizinischen Zuständen, Beschwerden oder Krankheiten erhalten haben. Wir müssen betonen, dass jede Form der körperlichen Einführung dieser Produkte in Menschen oder Tiere gesetzlich strikt untersagt ist. Es ist unerlässlich, sich an diese Richtlinien zu halten, um die Einhaltung rechtlicher und ethischer Standards in Forschung und Experiment zu gewährleisten.