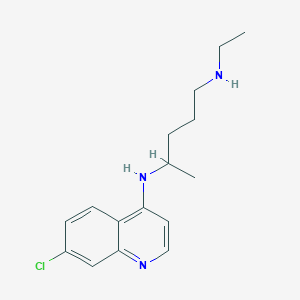
Desethylchloroquine
Übersicht
Beschreibung
Desethylchloroquin ist ein Metabolit von Chloroquin und Hydroxychloroquin, die als Antimalariamittel bekannt sind. Desethylchloroquin wurde auf seine pharmakologische Aktivität und seine Rolle im Metabolismus von Chloroquin und Hydroxychloroquin untersucht .
Vorbereitungsmethoden
Desethylchloroquin wird typischerweise durch Dealkylierung von Chloroquin synthetisiert. Dieser Prozess beinhaltet die Entfernung einer Ethylgruppe von Chloroquin, die hauptsächlich durch Cytochrom-P450-Enzyme wie CYP2C8, CYP3A4 und in geringerem Maße CYP2D6 vermittelt wird . Der Syntheseweg beinhaltet die Verwendung spezifischer Reagenzien und Bedingungen, um die gewünschte Dealkylierung zu erreichen.
In der industriellen Produktion wird häufig die Hochleistungsflüssigkeitschromatographie (HPLC) in Verbindung mit UV-Detektoren eingesetzt, um Chloroquin und seine Metaboliten, einschließlich Desethylchloroquin, in pharmazeutischen Produkten und biologischen Proben zu quantifizieren . Dieses Verfahren gewährleistet die Reinheit und Qualität der Verbindung für verschiedene Anwendungen.
Analyse Chemischer Reaktionen
Desethylchloroquin unterliegt verschiedenen Arten chemischer Reaktionen, darunter:
Oxidation: Diese Reaktion beinhaltet die Addition von Sauerstoff oder die Entfernung von Wasserstoff, die oft durch Oxidationsmittel gefördert wird.
Reduktion: Die Addition von Wasserstoff oder die Entfernung von Sauerstoff, typischerweise unter Verwendung von Reduktionsmitteln.
Substitution: Austausch einer funktionellen Gruppe durch eine andere, der unter verschiedenen Bedingungen stattfinden kann.
Zu den gängigen Reagenzien, die in diesen Reaktionen verwendet werden, gehören Oxidationsmittel wie Kaliumpermanganat und Reduktionsmittel wie Natriumborhydrid. Die Hauptprodukte, die aus diesen Reaktionen entstehen, hängen von den spezifischen Bedingungen und den verwendeten Reagenzien ab .
Wissenschaftliche Forschungsanwendungen
Desethylchloroquin hat eine breite Palette von Anwendungen in der wissenschaftlichen Forschung:
Wirkmechanismus
Desethylchloroquin übt seine Wirkung aus, indem es die lysosomale Aktivität und Autophagie beeinträchtigt. Es interagiert mit der Membranstabilität und verändert Signalwege und die transkriptionelle Aktivität, was zur Modulation der Zytokinproduktion führen kann . Der Wirkmechanismus der Verbindung ist noch im Entstehen, aber es ist bekannt, dass sie die Wirkung der Häm-Polymerase in Malariatropozoiten hemmt, wodurch die Umwandlung von Häm zu Hämozoin verhindert wird, wodurch der Parasit abgetötet wird .
Wirkmechanismus
Desethylchloroquine exerts its effects by interfering with lysosomal activity and autophagy. It interacts with membrane stability and alters signaling pathways and transcriptional activity, which can result in the modulation of cytokine production . The compound’s mechanism of action is still emerging, but it is known to inhibit the action of heme polymerase in malarial trophozoites, preventing the conversion of heme to hemazoin, thereby killing the parasite .
Vergleich Mit ähnlichen Verbindungen
Desethylchloroquin ist anderen 4-Aminoquinolinverbindungen wie Chloroquin und Hydroxychloroquin ähnlich. Es ist in seinem spezifischen Stoffwechselweg und seiner pharmakologischen Aktivität einzigartig. Ähnliche Verbindungen sind:
Chloroquin: Die Stammverbindung, von der Desethylchloroquin abgeleitet ist.
Hydroxychloroquin: Ein weiterer Metabolit von Chloroquin mit ähnlicher chemischer Struktur und Pharmakokinetik.
Bisdesethylchloroquin: Ein weiterer Metabolit, der durch weitere Dealkylierung von Desethylchloroquin gebildet wird.
Die Einzigartigkeit von Desethylchloroquin liegt in seinen spezifischen Wechselwirkungen mit Cytochrom-P450-Enzymen und seinem besonderen pharmakologischen Profil .
Eigenschaften
IUPAC Name |
4-N-(7-chloroquinolin-4-yl)-1-N-ethylpentane-1,4-diamine | |
---|---|---|
Source | PubChem | |
URL | https://pubchem.ncbi.nlm.nih.gov | |
Description | Data deposited in or computed by PubChem | |
InChI |
InChI=1S/C16H22ClN3/c1-3-18-9-4-5-12(2)20-15-8-10-19-16-11-13(17)6-7-14(15)16/h6-8,10-12,18H,3-5,9H2,1-2H3,(H,19,20) | |
Source | PubChem | |
URL | https://pubchem.ncbi.nlm.nih.gov | |
Description | Data deposited in or computed by PubChem | |
InChI Key |
MCYUUUTUAAGOOT-UHFFFAOYSA-N | |
Source | PubChem | |
URL | https://pubchem.ncbi.nlm.nih.gov | |
Description | Data deposited in or computed by PubChem | |
Canonical SMILES |
CCNCCCC(C)NC1=C2C=CC(=CC2=NC=C1)Cl | |
Source | PubChem | |
URL | https://pubchem.ncbi.nlm.nih.gov | |
Description | Data deposited in or computed by PubChem | |
Molecular Formula |
C16H22ClN3 | |
Source | PubChem | |
URL | https://pubchem.ncbi.nlm.nih.gov | |
Description | Data deposited in or computed by PubChem | |
DSSTOX Substance ID |
DTXSID201033929 | |
Record name | Desethylchloroquine | |
Source | EPA DSSTox | |
URL | https://comptox.epa.gov/dashboard/DTXSID201033929 | |
Description | DSSTox provides a high quality public chemistry resource for supporting improved predictive toxicology. | |
Molecular Weight |
291.82 g/mol | |
Source | PubChem | |
URL | https://pubchem.ncbi.nlm.nih.gov | |
Description | Data deposited in or computed by PubChem | |
Physical Description |
Solid | |
Record name | Desethylchloroquine | |
Source | Human Metabolome Database (HMDB) | |
URL | http://www.hmdb.ca/metabolites/HMDB0041870 | |
Description | The Human Metabolome Database (HMDB) is a freely available electronic database containing detailed information about small molecule metabolites found in the human body. | |
Explanation | HMDB is offered to the public as a freely available resource. Use and re-distribution of the data, in whole or in part, for commercial purposes requires explicit permission of the authors and explicit acknowledgment of the source material (HMDB) and the original publication (see the HMDB citing page). We ask that users who download significant portions of the database cite the HMDB paper in any resulting publications. | |
CAS No. |
1476-52-4 | |
Record name | (±)-Desethylchloroquine | |
Source | CAS Common Chemistry | |
URL | https://commonchemistry.cas.org/detail?cas_rn=1476-52-4 | |
Description | CAS Common Chemistry is an open community resource for accessing chemical information. Nearly 500,000 chemical substances from CAS REGISTRY cover areas of community interest, including common and frequently regulated chemicals, and those relevant to high school and undergraduate chemistry classes. This chemical information, curated by our expert scientists, is provided in alignment with our mission as a division of the American Chemical Society. | |
Explanation | The data from CAS Common Chemistry is provided under a CC-BY-NC 4.0 license, unless otherwise stated. | |
Record name | Desethylchloroquine | |
Source | ChemIDplus | |
URL | https://pubchem.ncbi.nlm.nih.gov/substance/?source=chemidplus&sourceid=0001476524 | |
Description | ChemIDplus is a free, web search system that provides access to the structure and nomenclature authority files used for the identification of chemical substances cited in National Library of Medicine (NLM) databases, including the TOXNET system. | |
Record name | 1476-52-4 | |
Source | DTP/NCI | |
URL | https://dtp.cancer.gov/dtpstandard/servlet/dwindex?searchtype=NSC&outputformat=html&searchlist=13254 | |
Description | The NCI Development Therapeutics Program (DTP) provides services and resources to the academic and private-sector research communities worldwide to facilitate the discovery and development of new cancer therapeutic agents. | |
Explanation | Unless otherwise indicated, all text within NCI products is free of copyright and may be reused without our permission. Credit the National Cancer Institute as the source. | |
Record name | Desethylchloroquine | |
Source | EPA DSSTox | |
URL | https://comptox.epa.gov/dashboard/DTXSID201033929 | |
Description | DSSTox provides a high quality public chemistry resource for supporting improved predictive toxicology. | |
Record name | MONOETHYL CHLOROQUINE | |
Source | FDA Global Substance Registration System (GSRS) | |
URL | https://gsrs.ncats.nih.gov/ginas/app/beta/substances/ZC9Z9XX2PD | |
Description | The FDA Global Substance Registration System (GSRS) enables the efficient and accurate exchange of information on what substances are in regulated products. Instead of relying on names, which vary across regulatory domains, countries, and regions, the GSRS knowledge base makes it possible for substances to be defined by standardized, scientific descriptions. | |
Explanation | Unless otherwise noted, the contents of the FDA website (www.fda.gov), both text and graphics, are not copyrighted. They are in the public domain and may be republished, reprinted and otherwise used freely by anyone without the need to obtain permission from FDA. Credit to the U.S. Food and Drug Administration as the source is appreciated but not required. | |
Record name | Desethylchloroquine | |
Source | Human Metabolome Database (HMDB) | |
URL | http://www.hmdb.ca/metabolites/HMDB0041870 | |
Description | The Human Metabolome Database (HMDB) is a freely available electronic database containing detailed information about small molecule metabolites found in the human body. | |
Explanation | HMDB is offered to the public as a freely available resource. Use and re-distribution of the data, in whole or in part, for commercial purposes requires explicit permission of the authors and explicit acknowledgment of the source material (HMDB) and the original publication (see the HMDB citing page). We ask that users who download significant portions of the database cite the HMDB paper in any resulting publications. | |
Retrosynthesis Analysis
AI-Powered Synthesis Planning: Our tool employs the Template_relevance Pistachio, Template_relevance Bkms_metabolic, Template_relevance Pistachio_ringbreaker, Template_relevance Reaxys, Template_relevance Reaxys_biocatalysis model, leveraging a vast database of chemical reactions to predict feasible synthetic routes.
One-Step Synthesis Focus: Specifically designed for one-step synthesis, it provides concise and direct routes for your target compounds, streamlining the synthesis process.
Accurate Predictions: Utilizing the extensive PISTACHIO, BKMS_METABOLIC, PISTACHIO_RINGBREAKER, REAXYS, REAXYS_BIOCATALYSIS database, our tool offers high-accuracy predictions, reflecting the latest in chemical research and data.
Strategy Settings
Precursor scoring | Relevance Heuristic |
---|---|
Min. plausibility | 0.01 |
Model | Template_relevance |
Template Set | Pistachio/Bkms_metabolic/Pistachio_ringbreaker/Reaxys/Reaxys_biocatalysis |
Top-N result to add to graph | 6 |
Feasible Synthetic Routes
A: While the precise mechanism of action remains incompletely understood, both chloroquine and Desethylchloroquine are believed to exert their antimalarial effects by interfering with the parasite's ability to detoxify heme, a toxic byproduct of hemoglobin digestion. [, , ]
ANone: The molecular formula of this compound is C17H22ClN3, and its molecular weight is 303.82 g/mol.
A: this compound, like its parent compound, is rapidly absorbed following oral administration. It exhibits extensive tissue distribution, with higher concentrations observed in the liver, spleen, kidneys, and lungs. [, , , , ]
A: The elimination half-life of this compound varies but is generally long, ranging from several days to weeks. This prolonged half-life contributes to its accumulation in the body during repeated administration. [, , , , , ]
A: Studies demonstrate that this compound can cross the placenta and is also excreted in breast milk, although at lower concentrations compared to chloroquine. [, ]
A: this compound can undergo further dealkylation to form bisthis compound (BDCQ). Both this compound and BDCQ are primarily eliminated through renal excretion. [, , ]
A: Studies indicate that hemoglobin genotype might influence this compound levels, with higher erythrocyte concentrations observed in individuals with sickle cell disease. []
A: this compound concentrations are generally higher in whole blood compared to plasma, suggesting its accumulation in blood cells. [, , ]
A: this compound exhibits comparable activity to chloroquine against chloroquine-sensitive Plasmodium falciparum strains. [, ]
A: Studies show that this compound is less effective than chloroquine against chloroquine-resistant strains, suggesting cross-resistance. [, , , ]
ANone: The provided research focuses primarily on this compound as a metabolite of chloroquine. No clinical trials specifically evaluating its efficacy as a standalone treatment are discussed.
A: While the provided research does not extensively discuss this compound's toxicity profile, its long half-life and potential for accumulation in the body highlight the need for careful monitoring during long-term chloroquine administration. [, , , ]
ANone: The research primarily focuses on short-term pharmacokinetics and antimalarial activity. Long-term effects of this compound exposure are not extensively addressed.
A: Various methods, including high-performance liquid chromatography (HPLC) with different detection techniques (fluorescence, diode array, mass spectrometry), capillary electrophoresis (CE), and thin-layer chromatography (TLC), have been employed for this compound quantification. [, , , , , , , , ]
A: Yes, analytical methods utilizing dried blood spots collected on filter paper have been developed for this compound measurement, facilitating sample collection and transportation in resource-limited settings. [, ]
A: Resistance mechanisms to this compound are likely similar to those observed for chloroquine, involving mutations in the PfCRT (Plasmodium falciparum chloroquine resistance transporter) and Pgh1 (P-glycoprotein homolog 1) genes. These mutations can alter drug accumulation within the parasite, reducing its efficacy. [, , ]
Haftungsausschluss und Informationen zu In-Vitro-Forschungsprodukten
Bitte beachten Sie, dass alle Artikel und Produktinformationen, die auf BenchChem präsentiert werden, ausschließlich zu Informationszwecken bestimmt sind. Die auf BenchChem zum Kauf angebotenen Produkte sind speziell für In-vitro-Studien konzipiert, die außerhalb lebender Organismen durchgeführt werden. In-vitro-Studien, abgeleitet von dem lateinischen Begriff "in Glas", beinhalten Experimente, die in kontrollierten Laborumgebungen unter Verwendung von Zellen oder Geweben durchgeführt werden. Es ist wichtig zu beachten, dass diese Produkte nicht als Arzneimittel oder Medikamente eingestuft sind und keine Zulassung der FDA für die Vorbeugung, Behandlung oder Heilung von medizinischen Zuständen, Beschwerden oder Krankheiten erhalten haben. Wir müssen betonen, dass jede Form der körperlichen Einführung dieser Produkte in Menschen oder Tiere gesetzlich strikt untersagt ist. Es ist unerlässlich, sich an diese Richtlinien zu halten, um die Einhaltung rechtlicher und ethischer Standards in Forschung und Experiment zu gewährleisten.