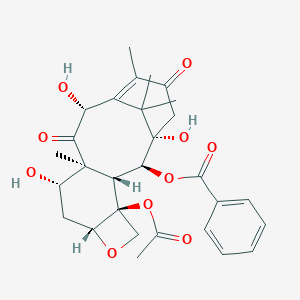
10-deacetyl-13-oxobaccatin III
Übersicht
Beschreibung
10-deacetyl-13-oxobaccatin III is a novel stable derivative of 10-Deacetylbaccatin III with antitumor and antimitotic activity . It is a white or off-white crystalline powder .
Synthesis Analysis
Baccatin III is an important precursor for the synthesis of the clinically important anticancer drug Taxol. A key enzyme of 10-deacetylbaccatin III-10-β-O-acetyltransferase (DBAT) catalyses the 10-deacetylbaccatin III into baccatin III in taxol biosynthesis . The crucial steps in the synthesis of 10-deacetyl-13-oxobaccatin III include the C14β hydroxylation of the corresponding 13-oxobaccatin III derivatives by oxaziridine-mediated electrophilic oxidation and the reduction of the C13 carbonyl group with sodium or alkylammonium borohydrides .Molecular Structure Analysis
The molecular formula of 10-deacetyl-13-oxobaccatin III is C29H34O10 . The molecular weight is 542.57 .Chemical Reactions Analysis
The chemical reactions involved in the synthesis of 10-deacetyl-13-oxobaccatin III include the C14β hydroxylation of the corresponding 13-oxobaccatin III derivatives by oxaziridine-mediated electrophilic oxidation and the reduction of the C13 carbonyl group with sodium or alkylammonium borohydrides .Physical And Chemical Properties Analysis
10-deacetyl-13-oxobaccatin III is a white or off-white crystalline powder . It is slightly soluble in chloroform, DMSO, and methanol .Wissenschaftliche Forschungsanwendungen
Precursor in Paclitaxel Biosynthesis
10-deacetyl-13-oxobaccatin III is a crucial precursor in the biosynthesis pathway of paclitaxel . Paclitaxel is recognized worldwide as one of the most effective cancer chemotherapeutic agents, and has been widely used in the clinical treatment of breast cancer, lung cancer, and colorectal cancers .
Source for Baccatin III Production
10-deacetyl-13-oxobaccatin III (10-DAB) can be used as a substrate for the chemical synthesis of Baccatin III . Baccatin III is another important precursor in the biosynthesis pathway of paclitaxel .
Biotransformation in Microbial Strains
Heterologous expression of 10-deacetylbaccatin III-10-O-acetyltransferase (TcDBAT) in microbial strains for biotransformation of 10-DAB is a promising alternative strategy for baccatin III production .
Extraction from Renewable Taxus Needles
Taxus needles are renewable and the content of 10-DAB is relatively high. They can be used as an effective source of the catalytic substrate 10-DAB . An integrated strategy based on 10-DAB extraction and in situ whole-cell biotransformation of renewable Taxus needles to produce Baccatin III has been developed .
Ultrasonic-assisted Extraction
The ultrasonic-assisted extraction of 10-deacetylbaccatin III from needles of Taxus baccata L. has been evaluated using ethanol and methanol as solvents . This extraction process has shown to yield reasonable amounts of 10-deacetylbaccatin III .
Cytotoxicity Against Cancer Cells
10-Deacetyl-13-oxobaccatin III shows cytotoxicity against A498, NCI-H226, A549, PC-3 cells, with inhibition rates of 29.7%, 49.2%, 43.9%, 65.3% respectively .
Safety and Hazards
The safety data sheet advises against using 10-deacetyl-13-oxobaccatin III for medicinal, household, or other uses . It is recommended to avoid dust formation, breathing mist, gas, or vapors, and contact with skin and eyes. Use personal protective equipment, wear chemical impermeable gloves, ensure adequate ventilation, remove all sources of ignition, evacuate personnel to safe areas, and keep people away from and upwind of spill/leak .
Zukünftige Richtungen
The enzymatically synthesized baccatin III (ESB III) has shown anticancer activities by inducing apoptotic cell death, which could further be used for the semisynthesis of taxol in the future . The literature suggests that 10-deacetylbaccatin III (10-DAB) can be easily obtained from plants and fungi for the semisynthesis of taxol. Further, different groups suggested that 10-DAB production can be increased through the optimization of culture conditions, genetic breeding, and metabolic engineering of the endophytic fungi and Taxus species plants .
Wirkmechanismus
Target of Action
10-Deacetyl-13-oxobaccatin III is a taxane , a class of compounds known for their anticancer properties. The primary targets of taxanes are microtubules, which are crucial components of the cell’s cytoskeleton . By binding to these structures, taxanes interfere with cell division, a process that is particularly active in cancer cells .
Mode of Action
10-Deacetyl-13-oxobaccatin III interacts with its targets, the microtubules, by binding to the β-tubulin subunit . This binding stabilizes the microtubules and prevents their depolymerization, a process necessary for cell division . As a result, the cell cycle is arrested, inhibiting the proliferation of cancer cells .
Biochemical Pathways
The action of 10-Deacetyl-13-oxobaccatin III affects the cell cycle pathway. By preventing the depolymerization of microtubules, it disrupts the formation of the mitotic spindle, a structure essential for chromosome segregation during cell division . This leads to cell cycle arrest at the G2/M phase, halting the proliferation of cancer cells .
Pharmacokinetics
Taxanes are generally poorly soluble in water and require formulation strategies for intravenous administration . They are widely distributed in the body and metabolized primarily in the liver . The bioavailability of 10-Deacetyl-13-oxobaccatin III would be influenced by these factors.
Result of Action
The result of 10-Deacetyl-13-oxobaccatin III’s action is the inhibition of cancer cell proliferation. It has shown cytotoxicity against various cancer cell lines, including A498, NCI-H226, A549, and PC-3 cells . The inhibition rates were 29.7%, 49.2%, 43.9%, and 65.3% respectively .
Action Environment
The action of 10-Deacetyl-13-oxobaccatin III, like other taxanes, can be influenced by various environmental factors. These include the pH and composition of the cellular environment, the presence of efflux pumps that can remove the drug from the cell, and the expression levels of microtubule-associated proteins that can affect drug binding . Additionally, genetic factors such as mutations in tubulin or other pathway components can influence the compound’s efficacy .
Eigenschaften
IUPAC Name |
[(1S,2S,3R,4S,7R,9S,10S,12R)-4-acetyloxy-1,9,12-trihydroxy-10,14,17,17-tetramethyl-11,15-dioxo-6-oxatetracyclo[11.3.1.03,10.04,7]heptadec-13-en-2-yl] benzoate | |
---|---|---|
Source | PubChem | |
URL | https://pubchem.ncbi.nlm.nih.gov | |
Description | Data deposited in or computed by PubChem | |
InChI |
InChI=1S/C29H34O10/c1-14-17(31)12-29(36)24(38-25(35)16-9-7-6-8-10-16)22-27(5,23(34)21(33)20(14)26(29,3)4)18(32)11-19-28(22,13-37-19)39-15(2)30/h6-10,18-19,21-22,24,32-33,36H,11-13H2,1-5H3/t18-,19+,21+,22-,24-,27+,28-,29+/m0/s1 | |
Source | PubChem | |
URL | https://pubchem.ncbi.nlm.nih.gov | |
Description | Data deposited in or computed by PubChem | |
InChI Key |
WMZBAMYUOYXRSF-RIFKXWPOSA-N | |
Source | PubChem | |
URL | https://pubchem.ncbi.nlm.nih.gov | |
Description | Data deposited in or computed by PubChem | |
Canonical SMILES |
CC1=C2C(C(=O)C3(C(CC4C(C3C(C(C2(C)C)(CC1=O)O)OC(=O)C5=CC=CC=C5)(CO4)OC(=O)C)O)C)O | |
Source | PubChem | |
URL | https://pubchem.ncbi.nlm.nih.gov | |
Description | Data deposited in or computed by PubChem | |
Isomeric SMILES |
CC1=C2[C@H](C(=O)[C@@]3([C@H](C[C@@H]4[C@]([C@H]3[C@@H]([C@@](C2(C)C)(CC1=O)O)OC(=O)C5=CC=CC=C5)(CO4)OC(=O)C)O)C)O | |
Source | PubChem | |
URL | https://pubchem.ncbi.nlm.nih.gov | |
Description | Data deposited in or computed by PubChem | |
Molecular Formula |
C29H34O10 | |
Source | PubChem | |
URL | https://pubchem.ncbi.nlm.nih.gov | |
Description | Data deposited in or computed by PubChem | |
DSSTOX Substance ID |
DTXSID80570271 | |
Record name | (5beta,7beta,10beta)-4-(Acetyloxy)-1,7,10-trihydroxy-9,13-dioxo-5,20-epoxytax-11-en-2-yl benzoate | |
Source | EPA DSSTox | |
URL | https://comptox.epa.gov/dashboard/DTXSID80570271 | |
Description | DSSTox provides a high quality public chemistry resource for supporting improved predictive toxicology. | |
Molecular Weight |
542.6 g/mol | |
Source | PubChem | |
URL | https://pubchem.ncbi.nlm.nih.gov | |
Description | Data deposited in or computed by PubChem | |
CAS RN |
92950-42-0 | |
Record name | (2aR,4S,4aS,6R,11S,12S,12aR,12bS)-12b-(Acetyloxy)-12-(benzoyloxy)-2a,4,4a,10,11,12,12a,12b-octahydro-4,6,11-trihydroxy-4a,8,13,13-tetramethyl-7,11-methano-1H-cyclodeca[3,4]benz[1,2-b]oxete-5,9(3H,6H)-dione | |
Source | CAS Common Chemistry | |
URL | https://commonchemistry.cas.org/detail?cas_rn=92950-42-0 | |
Description | CAS Common Chemistry is an open community resource for accessing chemical information. Nearly 500,000 chemical substances from CAS REGISTRY cover areas of community interest, including common and frequently regulated chemicals, and those relevant to high school and undergraduate chemistry classes. This chemical information, curated by our expert scientists, is provided in alignment with our mission as a division of the American Chemical Society. | |
Explanation | The data from CAS Common Chemistry is provided under a CC-BY-NC 4.0 license, unless otherwise stated. | |
Record name | (5beta,7beta,10beta)-4-(Acetyloxy)-1,7,10-trihydroxy-9,13-dioxo-5,20-epoxytax-11-en-2-yl benzoate | |
Source | EPA DSSTox | |
URL | https://comptox.epa.gov/dashboard/DTXSID80570271 | |
Description | DSSTox provides a high quality public chemistry resource for supporting improved predictive toxicology. | |
Retrosynthesis Analysis
AI-Powered Synthesis Planning: Our tool employs the Template_relevance Pistachio, Template_relevance Bkms_metabolic, Template_relevance Pistachio_ringbreaker, Template_relevance Reaxys, Template_relevance Reaxys_biocatalysis model, leveraging a vast database of chemical reactions to predict feasible synthetic routes.
One-Step Synthesis Focus: Specifically designed for one-step synthesis, it provides concise and direct routes for your target compounds, streamlining the synthesis process.
Accurate Predictions: Utilizing the extensive PISTACHIO, BKMS_METABOLIC, PISTACHIO_RINGBREAKER, REAXYS, REAXYS_BIOCATALYSIS database, our tool offers high-accuracy predictions, reflecting the latest in chemical research and data.
Strategy Settings
Precursor scoring | Relevance Heuristic |
---|---|
Min. plausibility | 0.01 |
Model | Template_relevance |
Template Set | Pistachio/Bkms_metabolic/Pistachio_ringbreaker/Reaxys/Reaxys_biocatalysis |
Top-N result to add to graph | 6 |
Feasible Synthetic Routes
Q & A
Q1: What is the significance of finding 10-deacetyl-13-oxobaccatin III in Taxus species?
A1: The discovery of 10-deacetyl-13-oxobaccatin III in Taxus sumatrana needles is significant because it adds to the growing library of taxoids isolated from these trees []. Taxoids, particularly paclitaxel (Taxol), are well-known for their anti-cancer activity. While 10-deacetyl-13-oxobaccatin III itself may not be as potent as paclitaxel, its discovery highlights the chemical diversity within Taxus species and the potential for finding new, potentially more effective or less toxic, anti-cancer compounds. Further research is needed to fully characterize its activity and potential therapeutic applications.
Q2: How does the chemical structure of 10-deacetyl-13-oxobaccatin III differ from other taxoids found in Taxus species?
A2: While the provided abstracts don't give detailed structural data for all compounds, they do mention that 10-deacetyl-13-oxobaccatin III was isolated alongside other taxoids like paclitaxel, cephalomannine, and baccatin III []. This suggests a structural similarity to these compounds. The name itself indicates key differences: "10-deacetyl" implies the absence of an acetyl group at the 10th carbon atom, and "13-oxo" indicates the presence of a ketone group at the 13th carbon. These structural variations can significantly influence the molecule's interactions with biological targets and consequently, its activity and potential therapeutic use.
Haftungsausschluss und Informationen zu In-Vitro-Forschungsprodukten
Bitte beachten Sie, dass alle Artikel und Produktinformationen, die auf BenchChem präsentiert werden, ausschließlich zu Informationszwecken bestimmt sind. Die auf BenchChem zum Kauf angebotenen Produkte sind speziell für In-vitro-Studien konzipiert, die außerhalb lebender Organismen durchgeführt werden. In-vitro-Studien, abgeleitet von dem lateinischen Begriff "in Glas", beinhalten Experimente, die in kontrollierten Laborumgebungen unter Verwendung von Zellen oder Geweben durchgeführt werden. Es ist wichtig zu beachten, dass diese Produkte nicht als Arzneimittel oder Medikamente eingestuft sind und keine Zulassung der FDA für die Vorbeugung, Behandlung oder Heilung von medizinischen Zuständen, Beschwerden oder Krankheiten erhalten haben. Wir müssen betonen, dass jede Form der körperlichen Einführung dieser Produkte in Menschen oder Tiere gesetzlich strikt untersagt ist. Es ist unerlässlich, sich an diese Richtlinien zu halten, um die Einhaltung rechtlicher und ethischer Standards in Forschung und Experiment zu gewährleisten.