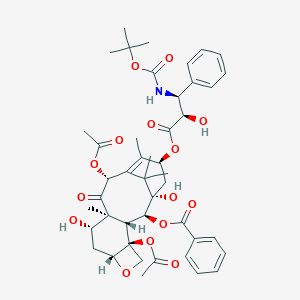
Docetaxel
Übersicht
Beschreibung
Docetaxel ist ein Chemotherapeutikum, das zur Behandlung verschiedener Krebsarten eingesetzt wird, darunter Brustkrebs, Kopf- und Halskrebs, Magenkrebs, Prostatakrebs und nicht-kleinzelliger Lungenkrebs . Es gehört zur Familie der Taxane und wirkt, indem es die normale Funktion von Mikrotubuli stört, wodurch die Zellteilung gestoppt wird . This compound wurde 1986 patentiert und 1995 für den medizinischen Gebrauch zugelassen . Es ist als Generikum erhältlich und steht auf der Liste der unentbehrlichen Arzneimittel der Weltgesundheitsorganisation .
Wissenschaftliche Forschungsanwendungen
Docetaxel hat eine breite Palette an Anwendungen in der wissenschaftlichen Forschung, insbesondere in den Bereichen Chemie, Biologie, Medizin und Industrie. In der Medizin wird es als Chemotherapeutikum zur Behandlung verschiedener Krebsarten eingesetzt . In der Chemie wird this compound auf seine einzigartigen chemischen Eigenschaften und Reaktionen untersucht . In der Biologie wird es verwendet, um die Mechanismen der Zellteilung und Apoptose zu untersuchen . In der Industrie werden this compound-beladene Nanoplattformen für die gezielte Wirkstoffabgabe und kontrollierte Freisetzung entwickelt, wodurch die Wirksamkeit verbessert und die Nebenwirkungen der Chemotherapie reduziert werden .
Wirkmechanismus
This compound übt seine Wirkungen aus, indem es die Montage von Mikrotubuli aus Tubulin-Dimeren fördert und ihre Depolymerisation hemmt . Diese Stabilisierung von Mikrotubuli stört die normale Funktion des Zytoskeletts, was zu einer Hemmung der Zellteilung und Induktion von Apoptose führt . This compound führt auch zur Phosphorylierung des Onkoproteins bcl-2, was die Apoptose in Krebszellen weiter fördert .
Wirkmechanismus
Target of Action
Docetaxel is a taxoid antineoplastic agent that primarily targets microtubules in cells . Microtubules are structures that help move chromosomes during mitosis (cell division) . This compound binds to microtubules with high affinity in a 1:1 stoichiometric ratio . This binding prevents cell division and promotes cell death .
Mode of Action
This compound interferes with the normal function of microtubule growth . Unlike drugs like colchicine that cause the depolymerization of microtubules, this compound hyper-stabilizes their structure . This destroys the cell’s ability to use its cytoskeleton in a flexible manner . This compound is two times more potent as an inhibitor of microtubule depolymerization compared to paclitaxel .
Biochemical Pathways
This compound’s action on microtubules affects the cell division process, leading to cell death . It also triggers the release of damage-associated molecular patterns (DAMPs) and pro-inflammatory cytokines, which activate the nuclear factor-kappa B (NF-κB) signaling pathway . This pathway is involved in the upregulation of PD-L1 in tumor cells .
Pharmacokinetics
This compound is subject to extensive metabolic conversion by the cytochrome P450 (CYP) 3A isoenzymes, which results in several pharmacologically inactive oxidation products . It is widely distributed in tissues with a mean volume of distribution of 74 L/m² after 100 mg/m², every 3 weeks . The mean total body clearance after this schedule is approximately 22 L/h/m², principally because of hepatic metabolism by the CYP3A4 system and biliary excretion into the feces .
Result of Action
The primary result of this compound’s action is the inhibition of cell division, leading to cell death . This cytotoxic activity is exhibited on various cancer cells, including breast, colorectal, lung, ovarian, gastric, renal, and prostate cancer cells .
Action Environment
The efficacy of this compound can be influenced by various environmental factors. For instance, its water solubility can affect its bioavailability and thus its efficacy . Additionally, the presence of drug-transporting proteins, notably P-glycoprotein, present on the bile canalicular membrane, can impact the elimination routes of this compound .
Biochemische Analyse
Biochemical Properties
Docetaxel reversibly binds to microtubulin with high affinity in a 1:1 stoichiometric ratio, allowing it to prevent cell division and promote cell death . Compared to paclitaxel, this compound is two times more potent as an inhibitor of microtubule depolymerization . This compound binds to microtubules but does not interact with dimeric tubulin .
Cellular Effects
This compound has been found to have greater cellular uptake and is retained longer intracellularly than paclitaxel, allowing this compound treatment to be effective with a smaller dose, leading to fewer and less severe adverse effects . It disrupts the normal function of microtubules and thereby stops cell division .
Molecular Mechanism
This compound works by disrupting the normal function of microtubules and thereby stopping cell division . It induces polymerization of tubulin, microtubule bundling in cells, formation of numerous abnormal mitotic asters . The cytotoxic effects of this compound are also severalfold greater than paclitaxel in vitro and in tumor xenografts .
Temporal Effects in Laboratory Settings
Traditionally limited by poor solubility and significant side effects, this compound’s therapeutic potential has been significantly enhanced through its incorporation into nanoplatforms, such as nanofibers and nanoparticles . This advancement offers targeted delivery, controlled release, and improved bioavailability, dramatically reducing systemic toxicity and enhancing patient outcomes .
Dosage Effects in Animal Models
In animal models, this compound has been administered at a dosage of 1 mg/kg on alternate days, as determined by a preliminary experiment . Significant arthritis index and knee joint circumference decrease were observed in the this compound group .
Metabolic Pathways
This compound is metabolized by the CYP3A4 isoenzyme . CYP3A5 also plays a role in the metabolism of this drug . In humans, this compound is metabolized by CYP3A4/5 into four metabolites: M1, M2, M3, and M4 .
Transport and Distribution
The intersection of nanotechnology and pharmacology has revolutionized the delivery and efficacy of chemotherapeutic agents, notably this compound . Nanoparticles enable precise drug delivery to tumor cells, minimizing damage to healthy tissues through sophisticated encapsulation methods such as nanoprecipitation and emulsion .
Subcellular Localization
The effects of this compound treatment on the expression and subcellular localization of ABC superfamily proteins have been studied . This compound treatment changes the expression level or subcellular localization of ABCB1, ABCC1, and ABCG2 .
Vorbereitungsmethoden
Docetaxel ist ein komplexes Diterpenoidmolekül und ein semisynthetisches Analogon von Paclitaxel . Die Herstellung von this compound umfasst verschiedene synthetische Routen und Reaktionsbedingungen. Ein häufiges Verfahren ist die Semisynthese aus 10-Deacetylbaccatin III, einer natürlich vorkommenden Verbindung, die aus den Nadeln der Europäischen Eibe (Taxus baccata) gewonnen wird . Die Synthese umfasst mehrere Schritte, darunter den Schutz und die Entschützung von funktionellen Gruppen, die Veresterung und die selektive Oxidation . Industrielle Produktionsverfahren umfassen häufig die Verwendung von hydrophilen Polymeren und Tensiden, um die Löslichkeit und Bioverfügbarkeit von this compound zu verbessern .
Analyse Chemischer Reaktionen
Docetaxel unterliegt verschiedenen chemischen Reaktionen, darunter Oxidations-, Reduktions- und Substitutionsreaktionen. Häufig verwendete Reagenzien in diesen Reaktionen sind Natriumlaurylsulfat, Eudragit L100 und andere hydrophile Polymere . Die Hauptprodukte, die aus diesen Reaktionen gebildet werden, sind typischerweise löslichere und bioverfügbarere Formen von this compound . Beispielsweise erhöht die Bildung von Einschlusskomplexen mit Alkylendiamin-modifizierten β-Cyclodextrinen die Löslichkeit und biologische Aktivität von this compound signifikant .
Vergleich Mit ähnlichen Verbindungen
Docetaxel ähnelt Paclitaxel, einem anderen Mitglied der Taxan-Familie, das in der Chemotherapie eingesetzt wird . Beide Verbindungen wirken, indem sie Mikrotubuli stabilisieren und die Zellteilung hemmen . This compound ist als Hemmer der Mikrotubuli-Depolymerisation potenter als Paclitaxel . Andere ähnliche Verbindungen sind Cabazitaxel und Larotaxel, die ebenfalls zur Taxan-Familie gehören und ähnliche Wirkmechanismen haben . This compound ist einzigartig in seiner höheren Affinität für Mikrotubuli und seiner Fähigkeit, einige Formen von Arzneimittelresistenz zu überwinden, die bei Paclitaxel beobachtet werden .
Biologische Aktivität
Docetaxel, a semi-synthetic derivative of paclitaxel, is widely used as a chemotherapeutic agent in the treatment of various cancers, including breast, lung, and prostate cancers. Its primary mechanism of action involves the stabilization of microtubules, which inhibits cell division and induces apoptosis in cancer cells. This article delves into the biological activity of this compound, highlighting its mechanisms, enhanced formulations, case studies, and research findings.
This compound exerts its effects primarily through the following mechanisms:
- Microtubule Stabilization : this compound binds to the β-subunit of tubulin in microtubules, preventing their depolymerization. This stabilization disrupts normal mitotic spindle function and leads to cell cycle arrest at the G2/M phase.
- Induction of Apoptosis : The stabilization of microtubules triggers signaling pathways that activate apoptotic processes. Studies have shown that this compound can increase the expression of pro-apoptotic proteins while decreasing anti-apoptotic factors .
- Antiangiogenic Properties : Recent studies indicate that this compound has significant antiangiogenic activity. For example, in a study involving metastatic prostate cancer models, this compound reduced small blood vessel formation and enhanced the effects of dexamethasone on angiogenesis .
Enhanced Formulations
Research has focused on improving the solubility and biological activity of this compound through novel formulations. These include:
- Inclusion Complexes : Cyclodextrin-based inclusion complexes have been developed to enhance the solubility and cytotoxicity of this compound. For instance, formulations with β-cyclodextrin derivatives showed improved water solubility by up to 5374 times and increased apoptosis rates in cancer cell lines compared to free this compound .
Formulation Type | Solubility Improvement | Apoptosis Induction |
---|---|---|
H1/DTX Complex | 216 times | Increased from 17.2% to 30.2% at 5 μg/mL |
H2/DTX Complex | 242 times | Increased from 19.0% to 31.0% at 10 μg/mL |
H3/DTX Complex | 253 times | Increased from 19.3% to 32.2% at 15 μg/mL |
Case Studies and Clinical Findings
Several clinical trials have evaluated the efficacy and safety of this compound in various cancer types:
- Breast Cancer : A study demonstrated that this compound significantly improved overall survival rates in patients with metastatic breast cancer when compared to standard therapies .
- Prostate Cancer : In combination with dexamethasone, this compound showed enhanced antiangiogenic effects in vivo, which correlated with improved patient outcomes in advanced prostate cancer cases .
- Lung Cancer : this compound has been used as a second-line treatment for non-small cell lung cancer (NSCLC), showing promising results in prolonging survival compared to best supportive care .
Research Findings
Recent research has highlighted various aspects of this compound's biological activity:
- Metabolite Analysis : A study isolated metabolites from human feces to assess their biological activity. Four oxidation products were identified, demonstrating reduced cytotoxicity compared to this compound itself, indicating potential for lower side effects while maintaining therapeutic efficacy .
- Toxicity Profiles : this compound is associated with myelosuppression and other adverse effects. However, research into modified dosing regimens has shown that careful management can mitigate these risks while maintaining efficacy .
Eigenschaften
IUPAC Name |
[(1S,2S,3R,4S,7R,9S,10S,12R,15S)-4,12-diacetyloxy-1,9-dihydroxy-15-[(2R,3S)-2-hydroxy-3-[(2-methylpropan-2-yl)oxycarbonylamino]-3-phenylpropanoyl]oxy-10,14,17,17-tetramethyl-11-oxo-6-oxatetracyclo[11.3.1.03,10.04,7]heptadec-13-en-2-yl] benzoate | |
---|---|---|
Source | PubChem | |
URL | https://pubchem.ncbi.nlm.nih.gov | |
Description | Data deposited in or computed by PubChem | |
InChI |
InChI=1S/C45H55NO15/c1-23-28(58-39(53)33(50)32(26-16-12-10-13-17-26)46-40(54)61-41(4,5)6)21-45(55)37(59-38(52)27-18-14-11-15-19-27)35-43(9,29(49)20-30-44(35,22-56-30)60-25(3)48)36(51)34(57-24(2)47)31(23)42(45,7)8/h10-19,28-30,32-35,37,49-50,55H,20-22H2,1-9H3,(H,46,54)/t28-,29-,30+,32-,33+,34+,35-,37-,43+,44-,45+/m0/s1 | |
Source | PubChem | |
URL | https://pubchem.ncbi.nlm.nih.gov | |
Description | Data deposited in or computed by PubChem | |
InChI Key |
LEMYAXKYCOBYOJ-OAGWZNDDSA-N | |
Source | PubChem | |
URL | https://pubchem.ncbi.nlm.nih.gov | |
Description | Data deposited in or computed by PubChem | |
Canonical SMILES |
CC1=C2C(C(=O)C3(C(CC4C(C3C(C(C2(C)C)(CC1OC(=O)C(C(C5=CC=CC=C5)NC(=O)OC(C)(C)C)O)O)OC(=O)C6=CC=CC=C6)(CO4)OC(=O)C)O)C)OC(=O)C | |
Source | PubChem | |
URL | https://pubchem.ncbi.nlm.nih.gov | |
Description | Data deposited in or computed by PubChem | |
Isomeric SMILES |
CC1=C2[C@H](C(=O)[C@@]3([C@H](C[C@@H]4[C@]([C@H]3[C@@H]([C@@](C2(C)C)(C[C@@H]1OC(=O)[C@@H]([C@H](C5=CC=CC=C5)NC(=O)OC(C)(C)C)O)O)OC(=O)C6=CC=CC=C6)(CO4)OC(=O)C)O)C)OC(=O)C | |
Source | PubChem | |
URL | https://pubchem.ncbi.nlm.nih.gov | |
Description | Data deposited in or computed by PubChem | |
Molecular Formula |
C45H55NO15 | |
Source | PubChem | |
URL | https://pubchem.ncbi.nlm.nih.gov | |
Description | Data deposited in or computed by PubChem | |
DSSTOX Substance ID |
DTXSID20925105 | |
Record name | 4,10-Bis(acetyloxy)-13-[(3-{[tert-butoxy(hydroxy)methylidene]amino}-2-hydroxy-3-phenylpropanoyl)oxy]-1,7-dihydroxy-9-oxo-5,20-epoxytax-11-en-2-yl benzoate | |
Source | EPA DSSTox | |
URL | https://comptox.epa.gov/dashboard/DTXSID20925105 | |
Description | DSSTox provides a high quality public chemistry resource for supporting improved predictive toxicology. | |
Molecular Weight |
849.9 g/mol | |
Source | PubChem | |
URL | https://pubchem.ncbi.nlm.nih.gov | |
Description | Data deposited in or computed by PubChem | |
CAS No. |
125354-16-7 | |
Record name | N-Debenzoyl-N-(tert-butoxycarbonyl)taxol | |
Source | ChemIDplus | |
URL | https://pubchem.ncbi.nlm.nih.gov/substance/?source=chemidplus&sourceid=0125354167 | |
Description | ChemIDplus is a free, web search system that provides access to the structure and nomenclature authority files used for the identification of chemical substances cited in National Library of Medicine (NLM) databases, including the TOXNET system. | |
Record name | 4,10-Bis(acetyloxy)-13-[(3-{[tert-butoxy(hydroxy)methylidene]amino}-2-hydroxy-3-phenylpropanoyl)oxy]-1,7-dihydroxy-9-oxo-5,20-epoxytax-11-en-2-yl benzoate | |
Source | EPA DSSTox | |
URL | https://comptox.epa.gov/dashboard/DTXSID20925105 | |
Description | DSSTox provides a high quality public chemistry resource for supporting improved predictive toxicology. | |
Retrosynthesis Analysis
AI-Powered Synthesis Planning: Our tool employs the Template_relevance Pistachio, Template_relevance Bkms_metabolic, Template_relevance Pistachio_ringbreaker, Template_relevance Reaxys, Template_relevance Reaxys_biocatalysis model, leveraging a vast database of chemical reactions to predict feasible synthetic routes.
One-Step Synthesis Focus: Specifically designed for one-step synthesis, it provides concise and direct routes for your target compounds, streamlining the synthesis process.
Accurate Predictions: Utilizing the extensive PISTACHIO, BKMS_METABOLIC, PISTACHIO_RINGBREAKER, REAXYS, REAXYS_BIOCATALYSIS database, our tool offers high-accuracy predictions, reflecting the latest in chemical research and data.
Strategy Settings
Precursor scoring | Relevance Heuristic |
---|---|
Min. plausibility | 0.01 |
Model | Template_relevance |
Template Set | Pistachio/Bkms_metabolic/Pistachio_ringbreaker/Reaxys/Reaxys_biocatalysis |
Top-N result to add to graph | 6 |
Feasible Synthetic Routes
Haftungsausschluss und Informationen zu In-Vitro-Forschungsprodukten
Bitte beachten Sie, dass alle Artikel und Produktinformationen, die auf BenchChem präsentiert werden, ausschließlich zu Informationszwecken bestimmt sind. Die auf BenchChem zum Kauf angebotenen Produkte sind speziell für In-vitro-Studien konzipiert, die außerhalb lebender Organismen durchgeführt werden. In-vitro-Studien, abgeleitet von dem lateinischen Begriff "in Glas", beinhalten Experimente, die in kontrollierten Laborumgebungen unter Verwendung von Zellen oder Geweben durchgeführt werden. Es ist wichtig zu beachten, dass diese Produkte nicht als Arzneimittel oder Medikamente eingestuft sind und keine Zulassung der FDA für die Vorbeugung, Behandlung oder Heilung von medizinischen Zuständen, Beschwerden oder Krankheiten erhalten haben. Wir müssen betonen, dass jede Form der körperlichen Einführung dieser Produkte in Menschen oder Tiere gesetzlich strikt untersagt ist. Es ist unerlässlich, sich an diese Richtlinien zu halten, um die Einhaltung rechtlicher und ethischer Standards in Forschung und Experiment zu gewährleisten.