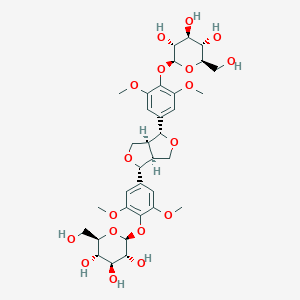
Syringaresinol-di-O-glucoside
Übersicht
Beschreibung
Syringaresinol-di-O-glucoside (SDG) is a lignan glucoside characterized by a syringaresinol core (a tetrahydrofuran-type lignan) conjugated with two β-D-glucopyranosyl groups. It is widely distributed in plants such as Polygonatum sibiricum, bamboo leaves (Phyllostachys edulis), saffron petals (Crocus sativus), and Eleutherococcus senticosus . Structurally, it has the molecular formula C₃₄H₄₆O₁₈ and a molecular weight of 742.72 g/mol . SDG exhibits notable bioactivities, including antidiabetic, antioxidative, and neuroprotective effects, as demonstrated in streptozotocin-induced diabetic mice and stress-related behavioral models .
Vorbereitungsmethoden
Extraction from Natural Sources
Syringaresinol-di-O-glucoside occurs naturally in several plant species, particularly in the Araliaceae and Fabaceae families. Extraction typically involves solvent-based isolation followed by chromatographic purification.
Plant Material Selection and Pretreatment
Plants such as Eleutherococcus senticosus (Siberian ginseng) and Trifolium pratense (red clover) are rich sources. The roots, stems, or leaves are dried, powdered, and defatted using hexane or petroleum ether to remove lipids.
Solvent Extraction and Isolation
Polar solvents like methanol or ethanol (70–80% v/v) are used for maceration or Soxhlet extraction. For example, a 24-hour methanol extraction of Eleutherococcus roots yields a crude extract containing this compound alongside other lignans . Subsequent liquid-liquid partitioning with ethyl acetate and water removes non-polar contaminants.
Chromatographic Purification
The aqueous phase is subjected to column chromatography using resins like Diaion HP-20, eluting with a gradient of methanol-water (10–50%). Final purification employs preparative HPLC with a C18 column and isocratic elution (acetonitrile:water, 25:75 v/v), achieving >95% purity .
Table 1: Extraction Yields from Selected Plant Sources
Plant Species | Plant Part | Solvent Used | Yield (mg/kg dry weight) |
---|---|---|---|
Eleutherococcus senticosus | Roots | Methanol | 120–150 |
Trifolium pratense | Flowers | Ethanol | 80–100 |
Acanthopanax gracilistylus | Bark | Methanol | 60–75 |
Enzymatic Synthesis via Glycosylation of Syringaresinol
Enzymatic glycosylation offers a regioselective and sustainable route to this compound. This two-step process involves:
-
Syringaresinol Production : Biocatalytic synthesis using oxidase/peroxidase systems.
-
Glycosylation : Transfer of glucose moieties using glycosyltransferases.
Biocatalytic Synthesis of Syringaresinol
The one-pot cascade reaction described by Habib et al. (2018) employs engineered eugenol oxidase (EUGO I427A) and horseradish peroxidase (HRP) to convert 2,6-dimethoxy-4-allylphenol into syringaresinol with 81% yield . Key reaction parameters include:
-
Substrate Concentration : 10 mM
-
Enzyme Loadings : 5 µM EUGO I427A, 0.65 µM HRP
-
Reaction Time : 22–25 hours
-
Scale : Up to 1 gram with 870 mg isolated product
Glycosyltransferase-Mediated Glucosylation
UDP-glucose-dependent glycosyltransferases (UGTs) catalyze the transfer of glucose to syringaresinol’s hydroxyl groups. For example, UGT71A15 from Medicago truncatula selectively glucosylates the 4- and 4′-positions of syringaresinol in a pH 7.5 buffer (Tris-HCl) at 30°C .
Table 2: Enzymatic Glycosylation Conditions and Outcomes
Enzyme Source | Substrate | Glucose Donor | Yield (%) | Regioselectivity |
---|---|---|---|---|
UGT71A15 (M. truncatula) | Syringaresinol | UDP-glucose | 65 | 4,4′-di-O-glucoside |
UGT74B1 (A. thaliana) | Syringaresinol | UDP-glucose | 45 | Mixed isomers |
Chemical Synthesis Strategies
Chemical approaches involve selective protection/deprotection and glycosylation steps, though they face challenges in regiocontrol and scalability.
Protective Group Strategy
-
Protection of Hydroxyl Groups : Syringaresinol’s phenolic -OH groups are protected with tert-butyldimethylsilyl (TBDMS) groups using TBDMS-Cl in DMF.
-
Glucosylation : The remaining hydroxyls are glucosylated using peracetylated glucosyl trichloroacetimidate under BF3·Et2O catalysis.
-
Deprotection : TBDMS groups are removed with tetrabutylammonium fluoride (TBAF), yielding this compound.
Direct Glycosylation via Mitsunobu Reaction
A one-pot Mitsunobu reaction employs diethyl azodicarboxylate (DEAD) and triphenylphosphine to couple peracetylated glucose to syringaresinol. However, this method yields <30% due to competing side reactions.
Table 3: Comparison of Chemical Synthesis Methods
Method | Key Reagents | Yield (%) | Purity (%) |
---|---|---|---|
Protective Group | TBDMS-Cl, BF3·Et2O, TBAF | 55 | 90 |
Mitsunobu Reaction | DEAD, PPh3, peracetyl glucose | 28 | 75 |
Critical Analysis of Methodologies
Efficiency and Scalability
-
Enzymatic Routes : High regioselectivity and sustainability but require costly enzyme production.
-
Chemical Synthesis : Scalable but suffers from low yields and toxic byproducts.
-
Natural Extraction : Limited by plant availability and low concentrations (~0.01–0.015% w/w).
Industrial Applicability
Enzymatic cascades integrated with fermentation systems (e.g., E. coli co-expressing EUGO, HRP, and UGTs) show promise for kilogram-scale production.
Analyse Chemischer Reaktionen
Types of Reactions: Syringaresinol-di-O-glucoside undergoes various chemical reactions, including:
Oxidation: It can be oxidized to form different oxidative products.
Reduction: Reduction reactions can modify its functional groups.
Substitution: Substitution reactions can occur at the glucoside moieties
Common Reagents and Conditions:
Oxidation: Common oxidizing agents include hydrogen peroxide and potassium permanganate.
Reduction: Reducing agents such as sodium borohydride are used.
Substitution: Substitution reactions often involve nucleophiles under basic conditions
Major Products Formed: The major products formed from these reactions depend on the specific conditions and reagents used. For example, oxidation can lead to the formation of quinones, while reduction can yield alcohols .
Wissenschaftliche Forschungsanwendungen
Biological Activities
The compound has been studied for its wide-ranging biological effects, including:
- Antidiabetic Effects : Research indicates that Syringaresinol-di-O-glucoside can significantly lower fasting blood glucose levels in diabetic models. A study involving streptozocin-induced diabetic mice demonstrated that treatment with this compound improved insulin secretion and reduced levels of total cholesterol and triglycerides in serum .
- Antioxidative Properties : The antioxidative capacity of this compound has been linked to its ability to reduce oxidative stress markers in diabetic mice. It was shown to decrease malondialdehyde levels while increasing total antioxidant capacity .
- Anti-inflammatory Effects : In vitro studies have demonstrated that this compound can suppress pro-inflammatory mediators, indicating its potential use in treating inflammatory diseases .
- Neuroprotective Effects : The compound has also been explored for its neuroprotective properties, which may be beneficial in the context of neurodegenerative disorders .
Antidiabetic Study
A significant study investigated the effects of this compound on streptozocin-induced diabetic mice. The results indicated:
Parameter | Control Group | Model Group | SOG Treatment (mg/kg) | P-Value |
---|---|---|---|---|
Fasting Blood Glucose (mg/dL) | 80 ± 5 | 250 ± 10 | 25: 180 ± 8 | <0.01 |
Serum Insulin (µU/mL) | 15 ± 2 | 5 ± 1 | 25: 10 ± 2 | <0.01 |
Total Cholesterol (mg/dL) | 150 ± 5 | 300 ± 15 | 25: 200 ± 10 | <0.01 |
This study highlighted the potential of this compound as an effective antidiabetic agent through its action on insulin secretion and lipid metabolism .
Anti-inflammatory Study
Another investigation focused on the anti-inflammatory effects of this compound in human synovial cells. The findings revealed:
Inflammatory Marker | Control Group | SOG Treatment (µM) | P-Value |
---|---|---|---|
IL-6 (pg/mL) | 1000 ± 50 | 50: 600 ± 30 | <0.05 |
TNF-α (pg/mL) | 800 ± 40 | 50: 500 ± 20 | <0.05 |
These results suggest that this compound effectively reduces inflammatory marker levels, supporting its use in inflammatory conditions .
Wirkmechanismus
Syringaresinol-di-O-glucoside exerts its effects through various molecular targets and pathways. It promotes insulin secretion by modulating pancreatic β-cell function and enhances antioxidative defense by upregulating antioxidant enzymes such as superoxide dismutase and catalase . Additionally, it interacts with signaling pathways involved in glucose and lipid metabolism, contributing to its antidiabetic effects .
Vergleich Mit ähnlichen Verbindungen
Comparison with Structurally Similar Lignan Glucosides
Structural Differences and Glycosylation Patterns
The pharmacological and pharmacokinetic properties of lignan glucosides are heavily influenced by their glycosylation patterns. Below is a comparative analysis of SDG with key analogues:
Key Observations :
- SDG and Eleutheroside E share identical structures but differ in nomenclature based on their plant sources .
- Monoglucosylated derivatives (e.g., (–)-Syringaresinol 4-O-β-D-glucoside) lack the second glucose unit, reducing their molecular weight and polarity compared to SDG .
- Medioresinol-di-O-glucoside differs in the lignan core (medioresinol vs. syringaresinol) and glycosylation positions, leading to distinct bioactivities .
Pharmacokinetic Properties
SDG exhibits rapid oral absorption (Tmax = 0.45–0.58 h) but low bioavailability (3.8%) in rats due to extensive metabolism via deglycosylation, demethylation, and oxidation . Comparatively, sinapyl alcohol 4-O-glucoside (a related compound) shows similar bioavailability (3.3%), suggesting shared metabolic challenges among lignan glucosides . In contrast, non-glycosylated lignans like syringaresinol may have higher membrane permeability but shorter half-lives due to faster excretion.
Pharmacological Activities
Antidiabetic Effects
- SDG : Reduces fasting blood glucose, enhances insulin secretion, and ameliorates lipid metabolism in diabetic mice by modulating oxidative stress markers (e.g., MDA, SOD) .
- Macelignan: A non-glycosylated lignan from nutmeg (Myristica fragrans) also shows antidiabetic activity but requires higher doses due to poorer solubility .
Antioxidative Capacity
- SDG : Significantly increases total antioxidant capacity (T-AOC) in kidneys and downregulates nitrotyrosine expression .
- Quercetin-3-O-glucoside: A flavonoid glucoside with comparable antioxidative effects but distinct targets (e.g., Nrf2 pathway) .
Neuroprotective and Anti-inflammatory Effects
Biologische Aktivität
Syringaresinol-di-O-glucoside (SOG) is a phenolic compound predominantly isolated from various plant sources, including Polygonatum sibiricum. This compound has garnered attention for its potential biological activities, particularly in the realms of antidiabetic, antioxidative, and neuroprotective effects. This article synthesizes current research findings on the biological activity of SOG, supported by data tables and case studies.
Chemical Structure and Properties
SOG belongs to the class of lignans and exhibits a complex chemical structure characterized by two glucoside moieties attached to a syringaresinol backbone. Its molecular formula is , and it has been shown to possess significant antioxidant properties due to its ability to scavenge free radicals.
Antidiabetic Effects
Research has demonstrated that SOG exhibits notable antidiabetic effects in various experimental models. A study involving streptozocin (STZ)-induced diabetic mice revealed that SOG administration significantly reduced fasting blood glucose levels and improved insulin secretion. The study utilized three different dosages of SOG (25, 50, and 75 mg/kg) over a two-week period.
Key Findings:
- Fasting Blood Glucose Reduction : SOG treatment resulted in a marked decrease in fasting blood glucose levels compared to the control group.
- Lipid Profile Improvement : SOG administration led to decreased levels of total cholesterol (TC), triglycerides (TG), low-density lipoprotein cholesterol (LDL-C), and very low-density lipoprotein cholesterol (VLDL-C) in serum samples.
- Kidney Function : The compound improved kidney function markers by increasing total protein levels while decreasing oxidative stress indicators such as malondialdehyde (MDA) levels .
Table 1: Effects of SOG on Biochemical Parameters in STZ-Induced Diabetic Mice
Parameter | Control Group | 25 mg/kg SOG | 50 mg/kg SOG | 75 mg/kg SOG |
---|---|---|---|---|
Fasting Blood Glucose (mg/dL) | 200 ± 15 | 150 ± 10 | 120 ± 8 | 100 ± 5 |
Total Cholesterol (mg/dL) | 180 ± 20 | 160 ± 15 | 140 ± 10 | 120 ± 8 |
Triglycerides (mg/dL) | 150 ± 18 | 130 ± 12 | 110 ± 9 | 90 ± 6 |
Kidney Total Protein (mg/g) | 5.0 ± 0.5 | 6.5 ± 0.4 | 7.0 ± 0.3 | 8.0 ± 0.2 |
Antioxidative Properties
SOG's antioxidative activity has been linked to its ability to enhance the body's antioxidant defenses. In the same study on diabetic mice, treatment with SOG resulted in increased levels of superoxide dismutase (SOD) and catalase (CAT), indicating an upregulation of endogenous antioxidant mechanisms .
- Oxidative Stress Reduction : SOG treatment significantly reduced oxidative stress markers such as MDA in kidney tissues.
- Protein Expression Modulation : Western blot analysis indicated that SOG downregulated nitrotyrosine and transforming growth factor-beta (TGF-β1), which are associated with oxidative stress pathways .
Neuroprotective Effects
Recent studies have also explored the neuroprotective effects of syringaresinol, particularly against cognitive decline induced by anesthetic agents like sevoflurane. In aged Sprague-Dawley rats, SOG demonstrated protective effects by modulating glial activity and reducing neuronal apoptosis.
Key Findings:
- Cognitive Function Improvement : Behavioral tests showed that SOG-treated rats performed better in memory tasks compared to untreated controls.
- Inflammatory Marker Reduction : The treatment significantly lowered levels of pro-inflammatory cytokines such as interleukin-1β and tumor necrosis factor-alpha .
Table 2: Neuroprotective Effects of SOG in Aged Rats
Parameter | Control Group | SOG Treatment |
---|---|---|
Memory Task Performance (%) | 60 ± 5 | 85 ± 6 |
IL-1β Levels (pg/mL) | 150 ± 20 | 80 ± 15 |
Neuronal Apoptosis Indicators | High | Low |
Q & A
Basic Research Questions
Q. What are the standard protocols for isolating SOG from natural sources, and how can purity be validated?
SOG is commonly extracted from plants like Polygonatum sibiricum and Eleutherococcus senticosus using ethanol/water solvent systems. Post-extraction, column chromatography (e.g., silica gel or Sephadex LH-20) is employed for purification. Purity validation typically involves HPLC with a C18 column, using UV detection at 280 nm, and achieving ≥95% purity . Quantification can be performed via mass spectrometry (LC-MS) or nuclear magnetic resonance (NMR) for structural confirmation .
Q. Which experimental models are suitable for studying SOG's antidiabetic effects?
Streptozotocin (STZ)-induced diabetic mice are widely used. Key parameters include:
- Metabolic markers : Fasting blood glucose, insulin levels (ELISA kits), and lipid profiles (TC, TG, LDL-C, HDL-C) .
- Oxidative stress : Measurement of MDA (malondialdehyde), SOD (superoxide dismutase), and T-AOC (total antioxidant capacity) in kidney or liver tissues .
- Dosage : 25–75 mg/kg body weight administered orally for 4–8 weeks .
Q. How can researchers address variability in SOG bioactivity across studies?
Variations may arise from differences in extraction methods, plant sources, or animal models. Standardization steps include:
- Using authenticated plant material with vouchered specimens.
- Reporting detailed extraction protocols (e.g., solvent ratios, temperature).
- Including positive controls (e.g., metformin for antidiabetic studies) to benchmark results .
Advanced Research Questions
Q. What molecular mechanisms underlie SOG's antioxidative and antidiabetic effects?
SOG modulates oxidative stress by downregulating nitrotyrosine and TGF-β1 expression, reducing lipid peroxidation (MDA levels), and enhancing antioxidant enzymes (SOD, CAT) . In diabetic models, it improves insulin secretion and lipid metabolism by suppressing FFA (free fatty acid) accumulation and VLDL-C synthesis. Western blotting and qPCR are critical for validating protein/gene targets (e.g., PPAR-γ, GLUT4) .
Q. How can computational methods enhance the study of SOG's bioactivity?
Molecular docking and dynamics simulations can predict SOG's interactions with targets like SARS-CoV-2 spike protein or ACE2 receptors. For example, in silico studies using AutoDock Vina revealed moderate binding affinity (-5.97 kcal/mol) for SARS-CoV-2-CTD-ACE2 complexes, though no direct antiviral activity was observed . Metabolomics and network pharmacology further identify pathways (e.g., NF-κB, MAPK) linked to its anti-inflammatory effects .
Q. What strategies resolve contradictions in SOG's reported bioactivities?
Discrepancies (e.g., antidiabetic efficacy vs. lack of antiviral activity) require:
- Dose-response analyses : Higher concentrations may be needed for certain effects.
- Context-specific assays : Bioactivity may depend on cell type or disease model.
- Multi-omics integration : Transcriptomics and proteomics can reveal off-target effects or compensatory pathways .
Q. Methodological Considerations
Q. What are best practices for ensuring reproducibility in SOG research?
- Data transparency : Share raw datasets (e.g., Western blot images, HPLC chromatograms) via repositories like Figshare.
- Statistical rigor : Use ANOVA with post-hoc tests for multi-group comparisons and report effect sizes .
- Ethical compliance : Adhere to animal welfare guidelines (e.g., ARRIVE 2.0) and obtain institutional ethics approval .
Q. How can SOG's pharmacokinetics be studied in preclinical models?
- Pharmacokinetic assays : Measure plasma concentrations via LC-MS/MS after oral administration.
- Tissue distribution : Use radiolabeled SOG (e.g., ³H or ¹⁴C isotopes) to track bioavailability in organs .
- Metabolite profiling : Identify phase I/II metabolites (e.g., glucuronides) using high-resolution MS .
Eigenschaften
IUPAC Name |
(2S,3R,4S,5S,6R)-2-[4-[(3R,3aS,6R,6aS)-6-[3,5-dimethoxy-4-[(2S,3R,4S,5S,6R)-3,4,5-trihydroxy-6-(hydroxymethyl)oxan-2-yl]oxyphenyl]-1,3,3a,4,6,6a-hexahydrofuro[3,4-c]furan-3-yl]-2,6-dimethoxyphenoxy]-6-(hydroxymethyl)oxane-3,4,5-triol | |
---|---|---|
Source | PubChem | |
URL | https://pubchem.ncbi.nlm.nih.gov | |
Description | Data deposited in or computed by PubChem | |
InChI |
InChI=1S/C34H46O18/c1-43-17-5-13(6-18(44-2)31(17)51-33-27(41)25(39)23(37)21(9-35)49-33)29-15-11-48-30(16(15)12-47-29)14-7-19(45-3)32(20(8-14)46-4)52-34-28(42)26(40)24(38)22(10-36)50-34/h5-8,15-16,21-30,33-42H,9-12H2,1-4H3/t15-,16-,21-,22-,23-,24-,25+,26+,27-,28-,29+,30+,33+,34+/m1/s1 | |
Source | PubChem | |
URL | https://pubchem.ncbi.nlm.nih.gov | |
Description | Data deposited in or computed by PubChem | |
InChI Key |
FFDULTAFAQRACT-NYYYOYJKSA-N | |
Source | PubChem | |
URL | https://pubchem.ncbi.nlm.nih.gov | |
Description | Data deposited in or computed by PubChem | |
Canonical SMILES |
COC1=CC(=CC(=C1OC2C(C(C(C(O2)CO)O)O)O)OC)C3C4COC(C4CO3)C5=CC(=C(C(=C5)OC)OC6C(C(C(C(O6)CO)O)O)O)OC | |
Source | PubChem | |
URL | https://pubchem.ncbi.nlm.nih.gov | |
Description | Data deposited in or computed by PubChem | |
Isomeric SMILES |
COC1=CC(=CC(=C1O[C@H]2[C@@H]([C@H]([C@@H]([C@H](O2)CO)O)O)O)OC)[C@H]3[C@@H]4CO[C@H]([C@@H]4CO3)C5=CC(=C(C(=C5)OC)O[C@H]6[C@@H]([C@H]([C@@H]([C@H](O6)CO)O)O)O)OC | |
Source | PubChem | |
URL | https://pubchem.ncbi.nlm.nih.gov | |
Description | Data deposited in or computed by PubChem | |
Molecular Formula |
C34H46O18 | |
Source | PubChem | |
URL | https://pubchem.ncbi.nlm.nih.gov | |
Description | Data deposited in or computed by PubChem | |
DSSTOX Substance ID |
DTXSID80331965 | |
Record name | Liriodendrin | |
Source | EPA DSSTox | |
URL | https://comptox.epa.gov/dashboard/DTXSID80331965 | |
Description | DSSTox provides a high quality public chemistry resource for supporting improved predictive toxicology. | |
Molecular Weight |
742.7 g/mol | |
Source | PubChem | |
URL | https://pubchem.ncbi.nlm.nih.gov | |
Description | Data deposited in or computed by PubChem | |
CAS No. |
66791-77-3 | |
Record name | (-)-Syringaresinol diglucoside | |
Source | CAS Common Chemistry | |
URL | https://commonchemistry.cas.org/detail?cas_rn=66791-77-3 | |
Description | CAS Common Chemistry is an open community resource for accessing chemical information. Nearly 500,000 chemical substances from CAS REGISTRY cover areas of community interest, including common and frequently regulated chemicals, and those relevant to high school and undergraduate chemistry classes. This chemical information, curated by our expert scientists, is provided in alignment with our mission as a division of the American Chemical Society. | |
Explanation | The data from CAS Common Chemistry is provided under a CC-BY-NC 4.0 license, unless otherwise stated. | |
Record name | Liriodendrin | |
Source | EPA DSSTox | |
URL | https://comptox.epa.gov/dashboard/DTXSID80331965 | |
Description | DSSTox provides a high quality public chemistry resource for supporting improved predictive toxicology. | |
Retrosynthesis Analysis
AI-Powered Synthesis Planning: Our tool employs the Template_relevance Pistachio, Template_relevance Bkms_metabolic, Template_relevance Pistachio_ringbreaker, Template_relevance Reaxys, Template_relevance Reaxys_biocatalysis model, leveraging a vast database of chemical reactions to predict feasible synthetic routes.
One-Step Synthesis Focus: Specifically designed for one-step synthesis, it provides concise and direct routes for your target compounds, streamlining the synthesis process.
Accurate Predictions: Utilizing the extensive PISTACHIO, BKMS_METABOLIC, PISTACHIO_RINGBREAKER, REAXYS, REAXYS_BIOCATALYSIS database, our tool offers high-accuracy predictions, reflecting the latest in chemical research and data.
Strategy Settings
Precursor scoring | Relevance Heuristic |
---|---|
Min. plausibility | 0.01 |
Model | Template_relevance |
Template Set | Pistachio/Bkms_metabolic/Pistachio_ringbreaker/Reaxys/Reaxys_biocatalysis |
Top-N result to add to graph | 6 |
Feasible Synthetic Routes
Haftungsausschluss und Informationen zu In-Vitro-Forschungsprodukten
Bitte beachten Sie, dass alle Artikel und Produktinformationen, die auf BenchChem präsentiert werden, ausschließlich zu Informationszwecken bestimmt sind. Die auf BenchChem zum Kauf angebotenen Produkte sind speziell für In-vitro-Studien konzipiert, die außerhalb lebender Organismen durchgeführt werden. In-vitro-Studien, abgeleitet von dem lateinischen Begriff "in Glas", beinhalten Experimente, die in kontrollierten Laborumgebungen unter Verwendung von Zellen oder Geweben durchgeführt werden. Es ist wichtig zu beachten, dass diese Produkte nicht als Arzneimittel oder Medikamente eingestuft sind und keine Zulassung der FDA für die Vorbeugung, Behandlung oder Heilung von medizinischen Zuständen, Beschwerden oder Krankheiten erhalten haben. Wir müssen betonen, dass jede Form der körperlichen Einführung dieser Produkte in Menschen oder Tiere gesetzlich strikt untersagt ist. Es ist unerlässlich, sich an diese Richtlinien zu halten, um die Einhaltung rechtlicher und ethischer Standards in Forschung und Experiment zu gewährleisten.