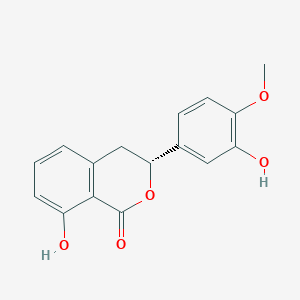
Phyllodulcin
Übersicht
Beschreibung
Phyllodulcin is a dihydroisocoumarin found in Hydrangea macrophylla and Hydrangea serrata. It is a sweetener 400–800 times sweeter than sugar .
Synthesis Analysis
Phyllodulcin was structurally identified using an LC/MS system. Hydrangea leaves were processed by either hand rolling or mechanical blending, by exposing them at different drying temperatures (25 and 70 °C), and even by inducing bioconversion in leaf tissue .Molecular Structure Analysis
The molecular formula of Phyllodulcin is C16H14O5. Its IUPAC name is (3 R )-8-hydroxy-3- (3-hydroxy-4-methoxyphenyl)-3,4-dihydroisochromen-1-one .Chemical Reactions Analysis
The establishment of efficient bioconversion, extraction, and isolation processes for the production of phyllodulcin from sweet hydrangea leaves has been studied. Traditional hand rolling and drying at 70 °C significantly increased phyllodulcin accumulation in the leaves .Physical And Chemical Properties Analysis
Phyllodulcin has a molecular weight of 286.28 g/mol. It is a hydroxybenzoic acid .Wissenschaftliche Forschungsanwendungen
Antitumor and Anti-inflammatory Activities
Research has focused on the antitumor and anti-inflammatory activities of Phyllodulcin. It has been found to be effective in these areas, particularly constituents of H. macrophyla, which includes Phyllodulcin .
Natural Sweetener with Health Benefits
In Japan, Phyllodulcin has been used since 1920 as a high potency, health-friendly sweetener. Its natural sweetness makes it a good alternative to synthetic sweeteners, with potential benefits for treating metabolic disorders .
Obesity and Metabolic Abnormalities Treatment
A study investigated whether Phyllodulcin could improve metabolic abnormalities in high-fat diet-induced obese mice, suggesting its potential as a natural treatment option for obesity and related metabolic issues .
Alzheimer’s Disease Treatment Potential
Recent research indicates that Phyllodulcin could inhibit Aβ aggregation and decompose pre-aggregated Aβ clumps, making it a potential candidate for treating Alzheimer’s disease both in cellular models and animal studies .
Wirkmechanismus
Target of Action
Phyllodulcin primarily targets the amyloid β (Aβ) protein, which is a major cause of Alzheimer’s disease . It also interacts with adrenocortical phosphodiesterase .
Mode of Action
Phyllodulcin inhibits the aggregation of Aβ and decomposes pre-existing Aβ clumps . It also acts as a non-selective phosphodiesterase inhibitor .
Biochemical Pathways
Phyllodulcin is synthesized from phenylalanine through a series of enzymatic reactions, leading to the formation of cinnamic acid, p-coumaric acid, and finally phyllodulcin . It affects the lipogenesis-related genes, including CCAAT/enhancer-binding protein α (C/EBPα), peroxisome proliferator activated receptor γ (PPARγ), and sterol regulatory element-binding protein-1C (SREBP-1c). It also upregulates fat browning-related genes, including PR domain containing 16 (Prdm16), uncoupling protein 1 (UCP1), and peroxisome proliferator-activated receptor γ coactivator 1-α (PGC-1α) .
Pharmacokinetics
Phyllodulcin is sparingly soluble in water . It can efficiently penetrate the blood-brain barrier and get uniformly distributed in the brain . More detailed studies on its ADME properties are needed.
Result of Action
Phyllodulcin reduces subcutaneous fat mass, levels of plasma lipids, triglycerides, total cholesterol, and low-density lipoprotein cholesterol. It improves the levels of leptin, adiponectin, and fasting blood glucose . In Alzheimer’s disease models, it reduces memory impairments caused by the accumulation of amyloid proteins .
Action Environment
Environmental factors such as diet can influence the action of phyllodulcin. For instance, in high-fat diet-induced obese mice, supplementation with phyllodulcin led to significant metabolic improvements
Zukünftige Richtungen
Eigenschaften
IUPAC Name |
(3R)-8-hydroxy-3-(3-hydroxy-4-methoxyphenyl)-3,4-dihydroisochromen-1-one | |
---|---|---|
Source | PubChem | |
URL | https://pubchem.ncbi.nlm.nih.gov | |
Description | Data deposited in or computed by PubChem | |
InChI |
InChI=1S/C16H14O5/c1-20-13-6-5-9(7-12(13)18)14-8-10-3-2-4-11(17)15(10)16(19)21-14/h2-7,14,17-18H,8H2,1H3/t14-/m1/s1 | |
Source | PubChem | |
URL | https://pubchem.ncbi.nlm.nih.gov | |
Description | Data deposited in or computed by PubChem | |
InChI Key |
PBILBHLAPJTJOT-CQSZACIVSA-N | |
Source | PubChem | |
URL | https://pubchem.ncbi.nlm.nih.gov | |
Description | Data deposited in or computed by PubChem | |
Canonical SMILES |
COC1=C(C=C(C=C1)C2CC3=C(C(=CC=C3)O)C(=O)O2)O | |
Source | PubChem | |
URL | https://pubchem.ncbi.nlm.nih.gov | |
Description | Data deposited in or computed by PubChem | |
Isomeric SMILES |
COC1=C(C=C(C=C1)[C@H]2CC3=C(C(=CC=C3)O)C(=O)O2)O | |
Source | PubChem | |
URL | https://pubchem.ncbi.nlm.nih.gov | |
Description | Data deposited in or computed by PubChem | |
Molecular Formula |
C16H14O5 | |
Source | PubChem | |
URL | https://pubchem.ncbi.nlm.nih.gov | |
Description | Data deposited in or computed by PubChem | |
DSSTOX Substance ID |
DTXSID10944103 | |
Record name | (R)-3,4-Dihydro-8-hydroxy-3-(3-hydroxy-4-methoxyphenyl)-1H-2-benzopyran-1-one | |
Source | EPA DSSTox | |
URL | https://comptox.epa.gov/dashboard/DTXSID10944103 | |
Description | DSSTox provides a high quality public chemistry resource for supporting improved predictive toxicology. | |
Molecular Weight |
286.28 g/mol | |
Source | PubChem | |
URL | https://pubchem.ncbi.nlm.nih.gov | |
Description | Data deposited in or computed by PubChem | |
Product Name |
Phyllodulcin | |
CAS RN |
21499-23-0 | |
Record name | Phyllodulcin | |
Source | CAS Common Chemistry | |
URL | https://commonchemistry.cas.org/detail?cas_rn=21499-23-0 | |
Description | CAS Common Chemistry is an open community resource for accessing chemical information. Nearly 500,000 chemical substances from CAS REGISTRY cover areas of community interest, including common and frequently regulated chemicals, and those relevant to high school and undergraduate chemistry classes. This chemical information, curated by our expert scientists, is provided in alignment with our mission as a division of the American Chemical Society. | |
Explanation | The data from CAS Common Chemistry is provided under a CC-BY-NC 4.0 license, unless otherwise stated. | |
Record name | Phyllodulcin | |
Source | ChemIDplus | |
URL | https://pubchem.ncbi.nlm.nih.gov/substance/?source=chemidplus&sourceid=0021499230 | |
Description | ChemIDplus is a free, web search system that provides access to the structure and nomenclature authority files used for the identification of chemical substances cited in National Library of Medicine (NLM) databases, including the TOXNET system. | |
Record name | (R)-3,4-Dihydro-8-hydroxy-3-(3-hydroxy-4-methoxyphenyl)-1H-2-benzopyran-1-one | |
Source | EPA DSSTox | |
URL | https://comptox.epa.gov/dashboard/DTXSID10944103 | |
Description | DSSTox provides a high quality public chemistry resource for supporting improved predictive toxicology. | |
Record name | PHYLLODULCIN | |
Source | FDA Global Substance Registration System (GSRS) | |
URL | https://gsrs.ncats.nih.gov/ginas/app/beta/substances/9DDW04R41V | |
Description | The FDA Global Substance Registration System (GSRS) enables the efficient and accurate exchange of information on what substances are in regulated products. Instead of relying on names, which vary across regulatory domains, countries, and regions, the GSRS knowledge base makes it possible for substances to be defined by standardized, scientific descriptions. | |
Explanation | Unless otherwise noted, the contents of the FDA website (www.fda.gov), both text and graphics, are not copyrighted. They are in the public domain and may be republished, reprinted and otherwise used freely by anyone without the need to obtain permission from FDA. Credit to the U.S. Food and Drug Administration as the source is appreciated but not required. | |
Retrosynthesis Analysis
AI-Powered Synthesis Planning: Our tool employs the Template_relevance Pistachio, Template_relevance Bkms_metabolic, Template_relevance Pistachio_ringbreaker, Template_relevance Reaxys, Template_relevance Reaxys_biocatalysis model, leveraging a vast database of chemical reactions to predict feasible synthetic routes.
One-Step Synthesis Focus: Specifically designed for one-step synthesis, it provides concise and direct routes for your target compounds, streamlining the synthesis process.
Accurate Predictions: Utilizing the extensive PISTACHIO, BKMS_METABOLIC, PISTACHIO_RINGBREAKER, REAXYS, REAXYS_BIOCATALYSIS database, our tool offers high-accuracy predictions, reflecting the latest in chemical research and data.
Strategy Settings
Precursor scoring | Relevance Heuristic |
---|---|
Min. plausibility | 0.01 |
Model | Template_relevance |
Template Set | Pistachio/Bkms_metabolic/Pistachio_ringbreaker/Reaxys/Reaxys_biocatalysis |
Top-N result to add to graph | 6 |
Feasible Synthetic Routes
Q & A
Q1: What is the molecular formula and weight of phyllodulcin?
A1: Phyllodulcin has a molecular formula of C16H14O5 and a molecular weight of 286.28 g/mol. [, ]
Q2: What are the key structural features of phyllodulcin?
A2: Phyllodulcin is characterized by a dihydroisocoumarin skeleton with a 3-(4-hydroxy-3-methoxyphenyl) substituent. It exists as two enantiomers due to the chiral center at the C-3 position. [, , ]
Q3: How does the structure of phyllodulcin relate to its sweetness?
A3: The sweet taste of phyllodulcin is attributed to its specific three-dimensional structure. Studies suggest that a partially-extended conformation of the molecule is crucial for its interaction with sweet taste receptors. This conformation allows for optimal binding with the receptor, leading to the perception of sweetness. []
Q4: Are there spectroscopic data available for phyllodulcin?
A4: Yes, spectroscopic data including IR, UV, NMR, and MS spectra have been used to characterize and identify phyllodulcin from various sources, including plant extracts and cultured cells. [, ]
Q5: What are the reported biological activities of phyllodulcin?
A5: Phyllodulcin has demonstrated various biological activities, including anti-diabetic [], anti-obesity [], neuroprotective [], anti-allergic [, ], antimicrobial [, ], and anti-inflammatory effects. []
Q6: How does phyllodulcin exert its anti-diabetic effects?
A6: Research suggests that phyllodulcin may improve blood glucose control by enhancing glucose uptake and regulating the expression of genes involved in glucose metabolism. In a study using diabetic mice, phyllodulcin supplementation significantly reduced fasting blood glucose and HbA1c levels. Additionally, it increased the expression of glucose transporter 4 (GLUT4), which facilitates glucose uptake into cells. []
Q7: What is the role of phyllodulcin in mitigating obesity?
A7: Phyllodulcin supplementation in high-fat diet-induced obese mice showed promising results in reducing subcutaneous fat mass and improving plasma lipid profiles. It also modulated the expression of genes involved in lipogenesis and fat browning in adipose tissue, suggesting its potential as an anti-obesity agent. []
Q8: How does phyllodulcin offer neuroprotection?
A8: Phyllodulcin exhibits protective effects against neuronal injury. In a study using a cellular model of cerebral ischemia-reperfusion injury, phyllodulcin significantly improved cell viability and reduced oxidative stress. It also protected mitochondria, which are crucial for cellular energy production and are highly susceptible to damage during ischemia-reperfusion. []
Q9: What are the mechanisms behind the anti-allergic effects of phyllodulcin?
A9: Studies have shown that phyllodulcin, alongside other dihydroisocoumarins from Hydrangea macrophylla var. thunbergii, can inhibit allergic reactions. In particular, these compounds demonstrated significant inhibition of the passive cutaneous anaphylaxis (PCA) reaction in rats, highlighting their potential in managing allergic conditions. []
Q10: Can you elaborate on the antimicrobial properties of phyllodulcin?
A10: Phyllodulcin exhibits antifungal activity against various fungal species. Notably, it has shown promising activity against oral bacteria. This antifungal property makes it a potential candidate for developing novel antimicrobial agents, particularly for oral health applications. []
Q11: What is the primary natural source of phyllodulcin?
A11: Phyllodulcin is primarily found in the leaves of Hydrangea macrophylla var. thunbergii, commonly known as "Amacha" in Japan. This plant has a long history of use as a natural sweetener and traditional medicine in East Asia. [, , ]
Q12: Are there alternative methods for producing phyllodulcin?
A12: Yes, apart from extraction from plant material, phyllodulcin can be produced through chemical synthesis. Several synthetic routes have been developed, offering alternative approaches to obtain this compound. [, , ]
Q13: What are the potential applications of phyllodulcin?
A13: Due to its sweet taste and potential health benefits, phyllodulcin holds promise for applications in various industries:
- Food and Beverage: As a natural, low-calorie sweetener, it can be used in beverages, confectionery, and other food products as a healthier alternative to sugar. []
- Pharmaceuticals: Its diverse biological activities make it a potential candidate for developing drugs targeting diabetes, obesity, neurodegenerative diseases, and inflammatory conditions. [, , ]
- Cosmetics: Its antimicrobial and antioxidant properties could be beneficial in cosmetic formulations for skin and oral care products. [, ]
Q14: How does the sweetness of phyllodulcin compare to sucrose?
A14: Phyllodulcin is reported to be 400-800 times sweeter than sucrose, making it a high-intensity sweetener. []
Q15: What analytical methods are employed to quantify phyllodulcin?
A15: High-performance liquid chromatography (HPLC) coupled with various detectors, such as UV or mass spectrometry (MS), is commonly used for the quantitative analysis of phyllodulcin in plant materials and other matrices. [, , ]
Q16: What are the key factors influencing phyllodulcin content in Hydrangea macrophylla var. thunbergii?
A16: Factors such as cultivar type, cultivation conditions (soil pH, light exposure, temperature), harvesting time, and processing methods can significantly affect the phyllodulcin content in the plant. [, , ]
Q17: How is computational chemistry utilized in phyllodulcin research?
A17: Computational methods, including molecular mechanics, quantum mechanics, and molecular docking, are employed to study the conformational preferences of phyllodulcin, its interactions with receptors, and to design novel derivatives with improved sweetness or biological activity. [, ]
Q18: Are there any structure-activity relationship (SAR) studies available for phyllodulcin?
A18: Yes, extensive SAR studies have been conducted to understand the influence of structural modifications on the sweetness and other biological activities of phyllodulcin. These studies guide the development of novel phyllodulcin derivatives with tailored properties. [, , ]
Q19: What is known about the metabolic fate of phyllodulcin?
A19: Studies in rats have shown that phyllodulcin undergoes extensive metabolism, primarily in the liver and gut. Major metabolic pathways include sulfation, glucuronidation, and degradation by intestinal microflora. []
Q20: What are the challenges associated with the development of phyllodulcin as a commercial product?
A20: While promising, several challenges need to be addressed for the successful commercialization of phyllodulcin:
- Sustainable Production: Developing efficient and sustainable methods for large-scale production of phyllodulcin, whether through cultivation, metabolic engineering, or chemical synthesis, is crucial. [, ]
- Safety and Regulatory Approval: Comprehensive toxicological studies are needed to establish the safety profile of phyllodulcin for human consumption. Regulatory approval is essential for its use in food, beverages, and pharmaceuticals. [, ]
- Sensory Properties: Addressing potential off-tastes or aftertastes associated with phyllodulcin, common to many high-intensity sweeteners, is crucial for consumer acceptance. [, ]
Q21: What are the future directions for phyllodulcin research?
A21: Future research directions include:
- Mechanism of Action: Elucidating the precise molecular mechanisms underlying the various biological activities of phyllodulcin is crucial for developing targeted therapies. [, , ]
- Clinical Trials: Conducting well-designed clinical trials to evaluate the efficacy and safety of phyllodulcin in humans is essential for its application in disease management. []
- Novel Analogs: Designing and synthesizing novel phyllodulcin analogs with enhanced sweetness, improved pharmacokinetic properties, and reduced potential side effects is a promising area of research. [, ]
Haftungsausschluss und Informationen zu In-Vitro-Forschungsprodukten
Bitte beachten Sie, dass alle Artikel und Produktinformationen, die auf BenchChem präsentiert werden, ausschließlich zu Informationszwecken bestimmt sind. Die auf BenchChem zum Kauf angebotenen Produkte sind speziell für In-vitro-Studien konzipiert, die außerhalb lebender Organismen durchgeführt werden. In-vitro-Studien, abgeleitet von dem lateinischen Begriff "in Glas", beinhalten Experimente, die in kontrollierten Laborumgebungen unter Verwendung von Zellen oder Geweben durchgeführt werden. Es ist wichtig zu beachten, dass diese Produkte nicht als Arzneimittel oder Medikamente eingestuft sind und keine Zulassung der FDA für die Vorbeugung, Behandlung oder Heilung von medizinischen Zuständen, Beschwerden oder Krankheiten erhalten haben. Wir müssen betonen, dass jede Form der körperlichen Einführung dieser Produkte in Menschen oder Tiere gesetzlich strikt untersagt ist. Es ist unerlässlich, sich an diese Richtlinien zu halten, um die Einhaltung rechtlicher und ethischer Standards in Forschung und Experiment zu gewährleisten.