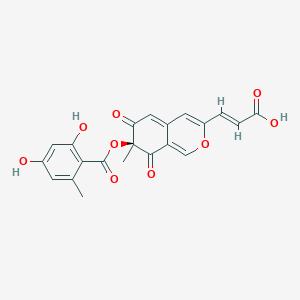
Mitorubrinsäure, (S)-
Übersicht
Beschreibung
Mitorubrinic acid, (S)- is a fungal metabolite primarily produced by the mold form of the fungus Penicillium marneffei. It is a yellow pigment that plays a significant role in the virulence of this thermal dimorphic fungus, which causes respiratory, skin, and systemic mycosis in China and Southeast Asia . The compound is part of the azaphilone class of fungal metabolites, known for their diverse biological activities .
Wissenschaftliche Forschungsanwendungen
Mitorubrinic acid has several scientific research applications:
Industry: It can be used as a natural pigment in various industrial applications.
Wirkmechanismus
Target of Action
Mitorubrinic acid, a member of the azaphilone family of natural products, has been found to have several biological targets. It induces the formation of chlamydospore-like cells in fungi and inhibits trypsin with an IC50 of 41 μmol/L . Its presumed dimer, diazaphilonic acid, inhibits Tth DNA polymerase with an IC50 of 2.6 μg/mL and is reported to completely inhibit MTI (human leukemia) telomerase activity .
Mode of Action
For instance, it inhibits trypsin, a serine protease involved in digestion, and Tth DNA polymerase, an enzyme essential for DNA replication .
Biochemical Pathways
Mitorubrinic acid is synthesized by two polyketide synthase (PKS) genes, named pks12 and pks11 . These genes are responsible for the biosynthesis of mitorubrinol and mitorubrinic acid, with pks12 and pks11 probably responsible for sequential use in the biosynthesis of these compounds .
Result of Action
The result of Mitorubrinic acid’s action is the inhibition of certain enzymes and the induction of specific cellular changes. For example, it inhibits trypsin, leading to potential effects on digestion . It also induces the formation of chlamydospore-like cells in fungi . Furthermore, it inhibits Tth DNA polymerase and MTI telomerase activity, which could have implications for cell proliferation and cancer .
Biochemische Analyse
Biochemical Properties
Mitorubrinic acid, (S)- plays a role in biochemical reactions, particularly in the biosynthesis of the yellow pigment in P. marneffei . It interacts with polyketide synthase genes, specifically PKS11 and PKS12, which are fungal non-reducing PKSs . These enzymes are likely responsible for the sequential use in the biosynthesis of mitorubrinol and mitorubrinic acid .
Cellular Effects
Mitorubrinic acid, (S)- has been observed to have significant effects on various types of cells and cellular processes. It is considered a virulence factor of P. marneffei, improving its intracellular survival in macrophages . This suggests that mitorubrinic acid, (S)- influences cell function, potentially impacting cell signaling pathways, gene expression, and cellular metabolism.
Molecular Mechanism
The molecular mechanism of action of Mitorubrinic acid, (S)- involves its interaction with PKS genes. PKS11 and PKS12 are likely responsible for the sequential use in the biosynthesis of mitorubrinol and mitorubrinic acid . This process may involve binding interactions with these biomolecules, potentially leading to changes in gene expression.
Temporal Effects in Laboratory Settings
The effects of Mitorubrinic acid, (S)- over time in laboratory settings are not fully understood. It is known that the yellow pigment composed of mitorubrinic acid and mitorubrinol is observed in the mold form of P. marneffei
Dosage Effects in Animal Models
The effects of Mitorubrinic acid, (S)- at different dosages in animal models are not well-documented. It has been observed that the survival of mice challenged with P. marneffei mutants was significantly better than those challenged with wild type P. marneffei
Metabolic Pathways
Mitorubrinic acid, (S)- is involved in the biosynthesis of the yellow pigment in P. marneffei, a process that likely involves the PKS11 and PKS12 enzymes This suggests that mitorubrinic acid, (S)- is part of a metabolic pathway involving these enzymes
Vorbereitungsmethoden
Synthetic Routes and Reaction Conditions: Mitorubrinic acid can be synthesized through polyketide synthase pathways. The genes pks11 and pks12 are responsible for its biosynthesis in Penicillium marneffei . The synthetic route involves the sequential use of these genes to produce mitorubrinol and mitorubrinic acid.
Industrial Production Methods: Industrial production of mitorubrinic acid is not well-documented, but it can be inferred that large-scale fermentation of Penicillium marneffei under controlled conditions could be a viable method. The fermentation process would involve optimizing the growth conditions to maximize the yield of mitorubrinic acid.
Analyse Chemischer Reaktionen
Types of Reactions: Mitorubrinic acid undergoes various chemical reactions, including oxidation, reduction, and substitution. These reactions are essential for modifying the compound for different applications.
Common Reagents and Conditions:
Oxidation: Common oxidizing agents such as potassium permanganate or hydrogen peroxide can be used.
Reduction: Reducing agents like sodium borohydride or lithium aluminum hydride are typically employed.
Substitution: Various nucleophiles can be used for substitution reactions, depending on the desired product.
Major Products: The major products formed from these reactions include derivatives of mitorubrinic acid with altered functional groups, which can have different biological activities and applications.
Vergleich Mit ähnlichen Verbindungen
- Lunatoic acid A
- Sclerotiorin
- Citrinin
Mitorubrinic acid stands out for its specific interactions with polyketide synthase genes and its significant role in the pathogenicity of Penicillium marneffei .
Biologische Aktivität
Mitorubrinic acid, specifically the (S)-enantiomer, is a member of the azaphilone family of natural products, primarily isolated from various fungal species. This compound has garnered attention due to its diverse biological activities, including antifungal properties, effects on cellular processes in fungi, and potential implications in virulence factors of pathogenic fungi.
Chemical Structure and Synthesis
Mitorubrinic acid features a complex structure characterized by an azaphilone nucleus. The total synthesis of this compound has been achieved through various methods, with significant steps involving the oxidative dearomatization of isocoumarin intermediates to construct the azaphilone framework. The synthetic route typically involves multiple steps to achieve the desired functionalization and yield .
Antifungal Properties
Mitorubrinic acid exhibits notable antifungal activity. It has been shown to induce the formation of chlamydospore-like cells in fungi, which are critical for fungal survival and reproduction. Additionally, it inhibits trypsin with an IC50 value of 41 μmol/L, indicating its potential as a protease inhibitor in various biological systems .
Virulence Factors in Pathogenic Fungi
Research has highlighted the role of mitorubrinic acid as a virulence factor in Penicillium marneffei, a significant pathogen in Southeast Asia. Studies demonstrate that mitorubrinic acid enhances the survival of this fungus within macrophages, suggesting that it may play a crucial role in its pathogenicity. The yellow pigment associated with P. marneffei is composed of mitorubrinic acid and mitorubrinol, both synthesized via polyketide synthase (PKS) genes identified as pks11 and pks12. Knockdown experiments have shown that mutants lacking these genes exhibit reduced virulence compared to wild-type strains .
Cultivation Studies
In cultivation studies, mitorubrinic acid was detected after 96 hours, with concentrations increasing significantly over extended periods. For instance, after 164 hours of cultivation, concentrations reached 1.08 g/dm³, demonstrating its biosynthetic efficiency within fungal cultures .
Interaction with Macrophages
In vitro studies using mouse macrophage cell lines revealed that mitorubrinic acid enhances intracellular survival rates of P. marneffei. This suggests that the compound not only aids in fungal growth but also provides a protective advantage against host immune responses .
Data Summary
Biological Activity | IC50 Value | Implications |
---|---|---|
Trypsin Inhibition | 41 μmol/L | Potential use as a protease inhibitor |
Induction of Chlamydospore Formation | N/A | Enhances fungal survival and reproduction |
Macrophage Survival Enhancement | N/A | Virulence factor in P. marneffei |
Eigenschaften
IUPAC Name |
(E)-3-[(7S)-7-(2,4-dihydroxy-6-methylbenzoyl)oxy-7-methyl-6,8-dioxoisochromen-3-yl]prop-2-enoic acid | |
---|---|---|
Source | PubChem | |
URL | https://pubchem.ncbi.nlm.nih.gov | |
Description | Data deposited in or computed by PubChem | |
InChI |
InChI=1S/C21H16O9/c1-10-5-12(22)8-15(23)18(10)20(28)30-21(2)16(24)7-11-6-13(3-4-17(25)26)29-9-14(11)19(21)27/h3-9,22-23H,1-2H3,(H,25,26)/b4-3+/t21-/m0/s1 | |
Source | PubChem | |
URL | https://pubchem.ncbi.nlm.nih.gov | |
Description | Data deposited in or computed by PubChem | |
InChI Key |
ZJIWQCFXEQSFGR-SHTLVRLNSA-N | |
Source | PubChem | |
URL | https://pubchem.ncbi.nlm.nih.gov | |
Description | Data deposited in or computed by PubChem | |
Canonical SMILES |
CC1=CC(=CC(=C1C(=O)OC2(C(=O)C=C3C=C(OC=C3C2=O)C=CC(=O)O)C)O)O | |
Source | PubChem | |
URL | https://pubchem.ncbi.nlm.nih.gov | |
Description | Data deposited in or computed by PubChem | |
Isomeric SMILES |
CC1=CC(=CC(=C1C(=O)O[C@]2(C(=O)C=C3C=C(OC=C3C2=O)/C=C/C(=O)O)C)O)O | |
Source | PubChem | |
URL | https://pubchem.ncbi.nlm.nih.gov | |
Description | Data deposited in or computed by PubChem | |
Molecular Formula |
C21H16O9 | |
Source | PubChem | |
URL | https://pubchem.ncbi.nlm.nih.gov | |
Description | Data deposited in or computed by PubChem | |
Molecular Weight |
412.3 g/mol | |
Source | PubChem | |
URL | https://pubchem.ncbi.nlm.nih.gov | |
Description | Data deposited in or computed by PubChem | |
CAS No. |
58958-07-9 | |
Record name | Mitorubrinic acid, (S)- | |
Source | ChemIDplus | |
URL | https://pubchem.ncbi.nlm.nih.gov/substance/?source=chemidplus&sourceid=0058958079 | |
Description | ChemIDplus is a free, web search system that provides access to the structure and nomenclature authority files used for the identification of chemical substances cited in National Library of Medicine (NLM) databases, including the TOXNET system. | |
Record name | MITORUBRINIC ACID, (S)- | |
Source | FDA Global Substance Registration System (GSRS) | |
URL | https://gsrs.ncats.nih.gov/ginas/app/beta/substances/651TW1SQXH | |
Description | The FDA Global Substance Registration System (GSRS) enables the efficient and accurate exchange of information on what substances are in regulated products. Instead of relying on names, which vary across regulatory domains, countries, and regions, the GSRS knowledge base makes it possible for substances to be defined by standardized, scientific descriptions. | |
Explanation | Unless otherwise noted, the contents of the FDA website (www.fda.gov), both text and graphics, are not copyrighted. They are in the public domain and may be republished, reprinted and otherwise used freely by anyone without the need to obtain permission from FDA. Credit to the U.S. Food and Drug Administration as the source is appreciated but not required. | |
Haftungsausschluss und Informationen zu In-Vitro-Forschungsprodukten
Bitte beachten Sie, dass alle Artikel und Produktinformationen, die auf BenchChem präsentiert werden, ausschließlich zu Informationszwecken bestimmt sind. Die auf BenchChem zum Kauf angebotenen Produkte sind speziell für In-vitro-Studien konzipiert, die außerhalb lebender Organismen durchgeführt werden. In-vitro-Studien, abgeleitet von dem lateinischen Begriff "in Glas", beinhalten Experimente, die in kontrollierten Laborumgebungen unter Verwendung von Zellen oder Geweben durchgeführt werden. Es ist wichtig zu beachten, dass diese Produkte nicht als Arzneimittel oder Medikamente eingestuft sind und keine Zulassung der FDA für die Vorbeugung, Behandlung oder Heilung von medizinischen Zuständen, Beschwerden oder Krankheiten erhalten haben. Wir müssen betonen, dass jede Form der körperlichen Einführung dieser Produkte in Menschen oder Tiere gesetzlich strikt untersagt ist. Es ist unerlässlich, sich an diese Richtlinien zu halten, um die Einhaltung rechtlicher und ethischer Standards in Forschung und Experiment zu gewährleisten.