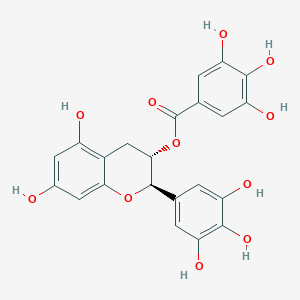
Gallocatechin-Gallat
Übersicht
Beschreibung
Paraherquamide A ist ein polycyclisches Oxindolalkaloid, das erstmals aus dem Pilz Penicillium paraherquei isoliert wurde. Es gehört zu einer einzigartigen Familie von prenylierten Indolalkaloiden, die für ihre komplexen Strukturen und signifikanten biologischen Aktivitäten bekannt sind . Paraherquamide A hat aufgrund seiner starken anthelmintischen Eigenschaften Aufmerksamkeit erlangt, was es zu einer wertvollen Verbindung im Bereich der Parasitologie macht .
Herstellungsmethoden
Synthetische Wege und Reaktionsbedingungen
Die Totalsynthese von Paraherquamide A ist aufgrund seiner komplizierten Struktur eine anspruchsvolle Aufgabe. Die erste stereogesteuerte Totalsynthese wurde in 48 chemischen Schritten erreicht, ausgehend von Ethylglycinat, Ethylacrylat und Vanillin . Wichtige Schritte in der Synthese beinhalten die regioselektive Prenylierung, Epoxidierung und ein Verfahren zur Bildung eines siebengliedrigen Rings . Die Synthese beinhaltet auch eine Reihe von Schutz- und Entschützungsschritten, Mannich-Reaktionen und selektiven Reduktionen .
Industrielle Produktionsmethoden
Die industrielle Produktion von Paraherquamide A basiert hauptsächlich auf der biologischen Fermentation. Der Pilz Penicillium sp. KWF31 wurde für die Hochleistungsproduktion von Paraherquamide A durch Response Surface Methodology optimiert . Wichtige Faktoren, die die Ausbeute beeinflussen, sind die Konzentrationen von löslicher Stärke, Glucose und Hefeextrakt . Unter optimalen Bedingungen kann der maximale Titer von Paraherquamide A 243,7 mg/L erreichen .
Wissenschaftliche Forschungsanwendungen
Neuroprotective Effects
Gallocatechin gallate exhibits notable neuroprotective properties, particularly against glutamate-induced neuronal cell death. A study demonstrated that gallocatechin gallate significantly reduced reactive oxygen species (ROS) levels and calcium influx in neuronal cells exposed to glutamate, which is known to cause oxidative stress and apoptosis. The findings indicated that gallocatechin gallate could inhibit the phosphorylation of signaling pathways involved in cell death, such as extracellular signal-regulated kinase and c-Jun N-terminal kinases, thereby protecting neuronal cells from damage .
Case Study: Neuroprotection Mechanism
- Study Design : HT22 neuronal cell line treated with glutamate and varying concentrations of gallocatechin gallate.
- Results :
- Reduced ROS levels by 50% at 100 μM concentration.
- Decreased nuclear condensation indicative of apoptosis.
- Inhibition of ERK and JNK phosphorylation.
Anticancer Properties
Research indicates that gallocatechin gallate possesses potent anticancer effects. In vitro studies have shown that it can inhibit the proliferation of various cancer cell lines. For instance, gallocatechin gallate demonstrated a significant reduction in the growth of human colorectal cancer cells (HCT-116), with an inhibition rate of approximately 79.2% at a concentration of 100 μM .
Data Table: Anticancer Efficacy
Compound | Concentration (μM) | Growth Inhibition (%) |
---|---|---|
Gallocatechin gallate | 100 | 79.2 |
Epigallocatechin gallate | 100 | 98.4 |
Catechin | 100 | 20.2 |
Cardiovascular Health
Gallocatechin gallate has been studied for its cardioprotective effects. A meta-analysis revealed that it significantly reduces myocardial infarct size and improves cardiac function in models of ischemia-reperfusion injury. These benefits are attributed to its antioxidant properties and ability to modulate lipid metabolism .
Case Study: Cardioprotection
- Study Design : Animal models subjected to myocardial ischemia-reperfusion injury.
- Results :
- Myocardial infarct size reduced by approximately 40%.
- Enhanced recovery of cardiac function post-injury.
Cognitive Enhancement
Recent studies have explored the cognitive-enhancing effects of gallocatechin gallate. A randomized controlled trial showed that a single dose of gallocatechin gallate-rich green tea extract improved cognitive performance in tasks requiring prefrontal cortex activation. Although the differences were not statistically significant, there was a trend towards improved performance compared to placebo .
Data Table: Cognitive Performance Results
Group | Pre-treatment Score | Post-treatment Score | Change (%) |
---|---|---|---|
Gallocatechin gallate | X1 | X2 | Y1 |
Placebo | X3 | X4 | Y2 |
Wirkmechanismus
Target of Action
Gallocatechin gallate (GCG) is a polyphenolic compound found in green tea that has been shown to interact with various targets. It has been reported to inhibit the early stage of infections, such as attachment, entry, and membrane fusion, by interfering with viral membrane proteins . GCG also influences cell surface growth factor receptors, mainly receptor tyrosine kinases, which participate in many processes including cell proliferation, survival, and angiogenesis .
Mode of Action
GCG exhibits both direct and indirect anti-infection effects. It directly inhibits early infection by interfering with the adsorption on host cells, inhibiting virus replication, and reducing bacterial biofilm formation and toxin release . Indirectly, GCG inhibits infection by regulating immune inflammation and antioxidation .
Biochemical Pathways
GCG has been shown to regulate multiple crucial cellular signaling pathways, including those mediated by EGFR, JAK-STAT, MAPKs, NF-κB, PI3K-AKT-mTOR, and others . Deregulation of these pathways is involved in the pathophysiology of various diseases. GCG’s anticancer activity is mediated by interfering with various cancer hallmarks .
Pharmacokinetics
The pharmacokinetics of GCG involve its absorption, distribution, metabolism, and excretion (ADME). The bioavailability of gcg is often discussed due to its poor absorption and rapid metabolism . Several studies have shown the ability to overcome poor bioavailability through nanotechnology-based strategies such as encapsulation, liposome, micelles, nanoparticles, and various other formulations .
Result of Action
GCG has been found to exhibit anti-proliferative, anti-angiogenic, and pro-apoptotic effects in numerous cancer cell lines and animal models . It has demonstrated the ability to interrupt various signaling pathways associated with cellular proliferation and division in different cancer types . Furthermore, GCG has shown neuroprotective effects against glutamate excitotoxicity .
Action Environment
The action of GCG can be influenced by various environmental factors. For instance, the composition of the gut microbiota can affect the action of GCG . Notably, GCG induced significant changes in the relative abundance of specific bacteria such as Lactobacillus, Faecalibaculum, and Bifidobacterium, which displayed high correlations with serum uric acid levels and uric acid-related gene expression .
Biochemische Analyse
Biochemical Properties
GCG interacts with various enzymes, proteins, and other biomolecules. It has been found to exhibit both pro- and antioxidant properties . GCG can function as an electron donor for peroxidase, resulting in the utilization of hydrogen peroxide . It also shows inhibitory effects on α-Glucosidase .
Cellular Effects
GCG has been shown to have significant effects on various types of cells and cellular processes. It has been found to exhibit the highest neuroprotective effect against glutamate excitotoxicity among all catechins . GCG can reduce nuclear condensation and the phosphorylation of extracellular signal-regulated kinase (ERK) and c-Jun N-terminal kinases (JNK) involved in cell death .
Molecular Mechanism
GCG exerts its effects at the molecular level through various mechanisms. It reduces the glutamate-induced increase in intracellular calcium . Furthermore, it inhibits the phosphorylation of ERK and JNK, leading to the effective suppression of neurocytotoxicity caused by glutamate in HT22 cells .
Temporal Effects in Laboratory Settings
The effects of GCG can change over time in laboratory settings. For instance, the amount of GCG does not differ in leaves of different stages, and the content is relatively low . In a high-temperature environment, an epimerization change is likely to occur, because heating results in the conversion from EGCG to GCG .
Dosage Effects in Animal Models
The effects of GCG vary with different dosages in animal models. For example, in a study on hyperuricemic mice induced by potassium oxonate, GCG treatment significantly reduced serum uric acid levels .
Metabolic Pathways
GCG is involved in various metabolic pathways. It has been found to promote the expression of uric acid secretion transporter genes while inhibiting the expression of uric acid reabsorption transporter genes in the kidney .
Vorbereitungsmethoden
Synthetic Routes and Reaction Conditions
The total synthesis of Paraherquamide A is a challenging process due to its intricate structure. The first stereocontrolled total synthesis was achieved in 48 chemical steps, starting from ethyl glycinate, ethyl acrylate, and vanillin . Key steps in the synthesis include regioselective prenylation, epoxidation, and a seven-membered ring-forming procedure . The synthesis also involves a series of protection and deprotection steps, Mannich reactions, and selective reductions .
Industrial Production Methods
Industrial production of Paraherquamide A primarily relies on biological fermentation. The fungus Penicillium sp. KWF31 has been optimized for high yield production of Paraherquamide A through response surface methodology . Key factors influencing the yield include the concentrations of soluble starch, glucose, and yeast extract . Under optimal conditions, the maximum titer of Paraherquamide A can reach 243.7 mg/L .
Analyse Chemischer Reaktionen
Paraherquamide A unterliegt verschiedenen chemischen Reaktionen, darunter:
Oxidation: Diese Reaktion kann verwendet werden, um die Oxindol-Einheit von Paraherquamide A zu modifizieren.
Reduktion: Selektive Reduktionen werden im synthetischen Weg eingesetzt, um die gewünschte Stereochemie zu erreichen.
Substitution: Prenylierung ist eine wichtige Substitutionsreaktion bei der Synthese von Paraherquamide A.
Häufig verwendete Reagenzien in diesen Reaktionen sind Oxidationsmittel wie DDQ, Reduktionsmittel wie Bäckerhefe und Schutzgruppen für selektive Reaktionen . Wichtige Produkte, die aus diesen Reaktionen entstehen, sind verschiedene Zwischenprodukte, die zur endgültigen Paraherquamide A-Struktur führen .
Vergleich Mit ähnlichen Verbindungen
Paraherquamide A gehört zu einer Familie von prenylierten Indolalkaloiden, zu denen Verbindungen wie:
- Brevianamide
- Marcfortine
- Asperparaline
- Stephacidine
- Notoamide
Was Paraherquamide A auszeichnet, ist sein einzigartiges Bicyclo[2.2.2]diazaoctan-Ringsystem, das vermutlich aus einer biologischen intramolekularen Diels-Alder-Cycloadditionsreaktion entsteht . Dieses strukturelle Merkmal trägt zu seinen starken biologischen Aktivitäten bei und macht es zu einer wertvollen Verbindung für weitere Forschung und Entwicklung.
Biologische Aktivität
Gallocatechin gallate (GCG) is a polyphenolic compound found primarily in green tea, recognized for its diverse biological activities. This article provides an in-depth exploration of GCG's biological effects, including its antioxidant, anti-inflammatory, neuroprotective, and anticancer properties, supported by various studies and data.
Chemical Structure and Properties
GCG is a gallate ester of gallocatechin, a type of catechin. Its molecular formula is CHO, and it features a phenolic structure that contributes to its biological activities.
Antioxidant Activity
GCG exhibits significant antioxidant properties, which are crucial for mitigating oxidative stress in cells. Various studies have measured its ability to scavenge free radicals and inhibit lipid peroxidation.
- Oxygen Radical Absorbance Capacity (ORAC) : GCG has been shown to possess high ORAC values, indicating its effectiveness in trapping peroxyl radicals. In a comparative study, GCG demonstrated superior antioxidant activity compared to other catechins like epicatechin gallate (ECG) and epigallocatechin gallate (EGCG) .
Compound | ORAC Value (μmol Trolox equivalents/l) |
---|---|
Gallocatechin Gallate | High |
Epicatechin Gallate | Moderate |
Epigallocatechin Gallate | Very High |
Neuroprotective Effects
Recent research highlights GCG's neuroprotective capabilities, particularly against glutamate-induced oxidative stress. A study demonstrated that GCG significantly reduced intracellular calcium levels and reactive oxygen species (ROS), thereby protecting neuronal cells from apoptosis.
- Mechanism of Action : GCG inhibits the phosphorylation of extracellular signal-regulated kinase (ERK) and c-Jun N-terminal kinases (JNK), which are involved in cell death pathways. This inhibition leads to reduced nuclear condensation and cell survival under oxidative stress conditions .
Anticancer Properties
GCG has been extensively studied for its anticancer effects across various cancer cell lines. It has shown promise in inhibiting tumor growth and inducing apoptosis.
- In Vitro Studies : In experiments involving human colorectal cancer cells (HCT-116), GCG demonstrated potent antiproliferative effects, with a significant reduction in cell viability at concentrations as low as 100 µM . The following table summarizes the antiproliferative effects of different catechins:
Compound | Inhibition at 100 µM (%) | Inhibition at 300 µM (%) |
---|---|---|
Gallocatechin Gallate | 79.2 | 98.4 |
Epigallocatechin Gallate | 98.4 | >99 |
Epicatechin Gallate | 11.6 | 20.3 |
Anti-inflammatory Effects
GCG also exhibits anti-inflammatory properties by modulating various signaling pathways involved in inflammation. It has been shown to inhibit the expression of cyclooxygenase-2 (COX-2), a key enzyme involved in the inflammatory response.
- Clinical Implications : These anti-inflammatory effects suggest potential therapeutic applications of GCG in conditions characterized by chronic inflammation, such as arthritis and cardiovascular diseases .
Case Studies and Clinical Research
- Neuroprotection Study : A clinical trial assessed the effects of GCG-rich green tea extract on brain function in healthy individuals. It was found that acute administration reduced activation in the dorsolateral prefrontal cortex during cognitive tasks, suggesting potential benefits for cognitive function .
- Cancer Prevention : Epidemiological studies have linked green tea consumption with lower cancer incidence rates. The high levels of catechins, particularly GCG, are believed to contribute significantly to these protective effects .
Eigenschaften
IUPAC Name |
[(2R,3S)-5,7-dihydroxy-2-(3,4,5-trihydroxyphenyl)-3,4-dihydro-2H-chromen-3-yl] 3,4,5-trihydroxybenzoate | |
---|---|---|
Source | PubChem | |
URL | https://pubchem.ncbi.nlm.nih.gov | |
Description | Data deposited in or computed by PubChem | |
InChI |
InChI=1S/C22H18O11/c23-10-5-12(24)11-7-18(33-22(31)9-3-15(27)20(30)16(28)4-9)21(32-17(11)6-10)8-1-13(25)19(29)14(26)2-8/h1-6,18,21,23-30H,7H2/t18-,21+/m0/s1 | |
Source | PubChem | |
URL | https://pubchem.ncbi.nlm.nih.gov | |
Description | Data deposited in or computed by PubChem | |
InChI Key |
WMBWREPUVVBILR-GHTZIAJQSA-N | |
Source | PubChem | |
URL | https://pubchem.ncbi.nlm.nih.gov | |
Description | Data deposited in or computed by PubChem | |
Canonical SMILES |
C1C(C(OC2=CC(=CC(=C21)O)O)C3=CC(=C(C(=C3)O)O)O)OC(=O)C4=CC(=C(C(=C4)O)O)O | |
Source | PubChem | |
URL | https://pubchem.ncbi.nlm.nih.gov | |
Description | Data deposited in or computed by PubChem | |
Isomeric SMILES |
C1[C@@H]([C@H](OC2=CC(=CC(=C21)O)O)C3=CC(=C(C(=C3)O)O)O)OC(=O)C4=CC(=C(C(=C4)O)O)O | |
Source | PubChem | |
URL | https://pubchem.ncbi.nlm.nih.gov | |
Description | Data deposited in or computed by PubChem | |
Molecular Formula |
C22H18O11 | |
Source | PubChem | |
URL | https://pubchem.ncbi.nlm.nih.gov | |
Description | Data deposited in or computed by PubChem | |
DSSTOX Substance ID |
DTXSID701336201 | |
Record name | Gallocatechin gallate | |
Source | EPA DSSTox | |
URL | https://comptox.epa.gov/dashboard/DTXSID701336201 | |
Description | DSSTox provides a high quality public chemistry resource for supporting improved predictive toxicology. | |
Molecular Weight |
458.4 g/mol | |
Source | PubChem | |
URL | https://pubchem.ncbi.nlm.nih.gov | |
Description | Data deposited in or computed by PubChem | |
CAS No. |
5127-64-0 | |
Record name | Gallocatechin gallate | |
Source | CAS Common Chemistry | |
URL | https://commonchemistry.cas.org/detail?cas_rn=5127-64-0 | |
Description | CAS Common Chemistry is an open community resource for accessing chemical information. Nearly 500,000 chemical substances from CAS REGISTRY cover areas of community interest, including common and frequently regulated chemicals, and those relevant to high school and undergraduate chemistry classes. This chemical information, curated by our expert scientists, is provided in alignment with our mission as a division of the American Chemical Society. | |
Explanation | The data from CAS Common Chemistry is provided under a CC-BY-NC 4.0 license, unless otherwise stated. | |
Record name | Gallocatechin gallate | |
Source | ChemIDplus | |
URL | https://pubchem.ncbi.nlm.nih.gov/substance/?source=chemidplus&sourceid=0005127640 | |
Description | ChemIDplus is a free, web search system that provides access to the structure and nomenclature authority files used for the identification of chemical substances cited in National Library of Medicine (NLM) databases, including the TOXNET system. | |
Record name | Gallocatechin gallate | |
Source | EPA DSSTox | |
URL | https://comptox.epa.gov/dashboard/DTXSID701336201 | |
Description | DSSTox provides a high quality public chemistry resource for supporting improved predictive toxicology. | |
Record name | GALLOCATECHIN GALLATE | |
Source | FDA Global Substance Registration System (GSRS) | |
URL | https://gsrs.ncats.nih.gov/ginas/app/beta/substances/0C056HB16M | |
Description | The FDA Global Substance Registration System (GSRS) enables the efficient and accurate exchange of information on what substances are in regulated products. Instead of relying on names, which vary across regulatory domains, countries, and regions, the GSRS knowledge base makes it possible for substances to be defined by standardized, scientific descriptions. | |
Explanation | Unless otherwise noted, the contents of the FDA website (www.fda.gov), both text and graphics, are not copyrighted. They are in the public domain and may be republished, reprinted and otherwise used freely by anyone without the need to obtain permission from FDA. Credit to the U.S. Food and Drug Administration as the source is appreciated but not required. | |
Record name | 3-Galloylgallocatechin | |
Source | Human Metabolome Database (HMDB) | |
URL | http://www.hmdb.ca/metabolites/HMDB0041598 | |
Description | The Human Metabolome Database (HMDB) is a freely available electronic database containing detailed information about small molecule metabolites found in the human body. | |
Explanation | HMDB is offered to the public as a freely available resource. Use and re-distribution of the data, in whole or in part, for commercial purposes requires explicit permission of the authors and explicit acknowledgment of the source material (HMDB) and the original publication (see the HMDB citing page). We ask that users who download significant portions of the database cite the HMDB paper in any resulting publications. | |
Retrosynthesis Analysis
AI-Powered Synthesis Planning: Our tool employs the Template_relevance Pistachio, Template_relevance Bkms_metabolic, Template_relevance Pistachio_ringbreaker, Template_relevance Reaxys, Template_relevance Reaxys_biocatalysis model, leveraging a vast database of chemical reactions to predict feasible synthetic routes.
One-Step Synthesis Focus: Specifically designed for one-step synthesis, it provides concise and direct routes for your target compounds, streamlining the synthesis process.
Accurate Predictions: Utilizing the extensive PISTACHIO, BKMS_METABOLIC, PISTACHIO_RINGBREAKER, REAXYS, REAXYS_BIOCATALYSIS database, our tool offers high-accuracy predictions, reflecting the latest in chemical research and data.
Strategy Settings
Precursor scoring | Relevance Heuristic |
---|---|
Min. plausibility | 0.01 |
Model | Template_relevance |
Template Set | Pistachio/Bkms_metabolic/Pistachio_ringbreaker/Reaxys/Reaxys_biocatalysis |
Top-N result to add to graph | 6 |
Feasible Synthetic Routes
Haftungsausschluss und Informationen zu In-Vitro-Forschungsprodukten
Bitte beachten Sie, dass alle Artikel und Produktinformationen, die auf BenchChem präsentiert werden, ausschließlich zu Informationszwecken bestimmt sind. Die auf BenchChem zum Kauf angebotenen Produkte sind speziell für In-vitro-Studien konzipiert, die außerhalb lebender Organismen durchgeführt werden. In-vitro-Studien, abgeleitet von dem lateinischen Begriff "in Glas", beinhalten Experimente, die in kontrollierten Laborumgebungen unter Verwendung von Zellen oder Geweben durchgeführt werden. Es ist wichtig zu beachten, dass diese Produkte nicht als Arzneimittel oder Medikamente eingestuft sind und keine Zulassung der FDA für die Vorbeugung, Behandlung oder Heilung von medizinischen Zuständen, Beschwerden oder Krankheiten erhalten haben. Wir müssen betonen, dass jede Form der körperlichen Einführung dieser Produkte in Menschen oder Tiere gesetzlich strikt untersagt ist. Es ist unerlässlich, sich an diese Richtlinien zu halten, um die Einhaltung rechtlicher und ethischer Standards in Forschung und Experiment zu gewährleisten.