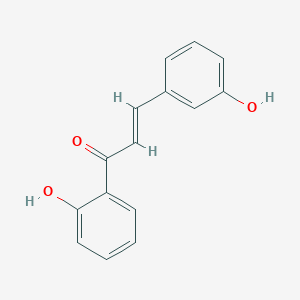
3,2'-Dihydroxychalcon
Übersicht
Beschreibung
3,2’-Dihydroxychalcone is a member of the chalcone family, which are flavonoid compounds characterized by the presence of two aromatic rings joined by a three-carbon α,β-unsaturated carbonyl system. This compound is known for its diverse biological activities and potential therapeutic applications .
Wissenschaftliche Forschungsanwendungen
3,2’-Dihydroxychalcone has a wide range of applications in scientific research:
Wirkmechanismus
Target of Action
3,2’-Dihydroxychalcone is known to interact with several targets. It has been found to activate bitter taste receptors, specifically hTAS2R14 and hTAS2R39 . These receptors play a crucial role in the perception of bitterness, which can influence food intake and metabolism.
Mode of Action
The interaction of 3,2’-Dihydroxychalcone with its targets leads to various changes. For instance, its activation of bitter taste receptors can trigger a cascade of intracellular events, leading to the perception of bitterness . .
Biochemical Pathways
3,2’-Dihydroxychalcone is implicated in several biochemical pathways. It has been suggested to have an inhibitory effect on the glycerol-3-phosphate dehydrogenase pathway in leishmania species . This pathway is crucial for energy production in these organisms, and its inhibition could potentially lead to their death.
Pharmacokinetics
Like other chalcones, it is expected to have good bioavailability due to its lipophilic nature .
Result of Action
The activation of bitter taste receptors by 3,2’-Dihydroxychalcone can lead to various molecular and cellular effects. For instance, it can influence food intake and metabolism, potentially contributing to weight management . Additionally, its inhibitory effect on the glycerol-3-phosphate dehydrogenase pathway in leishmania species could potentially be exploited for the treatment of leishmaniasis .
Biochemische Analyse
Biochemical Properties
3,2’-Dihydroxychalcone, like other chalcones, plays a significant role in biochemical reactions. It has been found to exhibit a moderate ability to reduce blood sugar . It also interacts with various enzymes, proteins, and other biomolecules, contributing to its wide range of bioactivities .
Cellular Effects
3,2’-Dihydroxychalcone has been shown to have multifaceted effects on various types of cells. It can modulate a number of cancer cell lines, inhibit pathological microorganisms and parasites, and control signaling molecules and cascades related to disease modification . It also has inhibitory effects on nitric oxide formation from LPS-stimulated murine microglial cells .
Molecular Mechanism
The molecular mechanism of action of 3,2’-Dihydroxychalcone involves its interactions at the molecular level. It has been suggested that it exerts its effects through binding interactions with biomolecules, enzyme inhibition or activation, and changes in gene expression .
Temporal Effects in Laboratory Settings
In laboratory settings, the effects of 3,2’-Dihydroxychalcone can change over time. For instance, it has been shown to exhibit a hypoglycemic effect comparable to that of metformin (an antidiabetic drug) in male mice with type 2 diabetes at doses of 200–300 mg/kg/day .
Metabolic Pathways
3,2’-Dihydroxychalcone is involved in the flavonoid biosynthesis pathway . Detailed information about the specific metabolic pathways that 3,2’-Dihydroxychalcone is involved in, including any enzymes or cofactors it interacts with, is currently limited.
Vorbereitungsmethoden
Synthetic Routes and Reaction Conditions: The most common method for synthesizing chalcones, including 3,2’-Dihydroxychalcone, is the Claisen-Schmidt condensation. This reaction involves the condensation of an aryl aldehyde with an aryl ketone in the presence of a base, typically an alcoholic alkali such as sodium hydroxide or potassium hydroxide . The reaction is usually carried out at room temperature or slightly elevated temperatures to facilitate the formation of the chalcone.
Industrial Production Methods: Industrial production of chalcones often involves similar synthetic routes but on a larger scale. The use of continuous flow reactors and optimization of reaction conditions can enhance yield and purity. Solvent extraction and recrystallization are commonly employed to purify the final product .
Analyse Chemischer Reaktionen
Types of Reactions: 3,2’-Dihydroxychalcone undergoes various chemical reactions, including:
Oxidation: This reaction can lead to the formation of quinones or other oxidized derivatives.
Reduction: Reduction reactions can convert the α,β-unsaturated carbonyl system to saturated systems.
Substitution: Electrophilic substitution reactions can occur on the aromatic rings, leading to various substituted chalcones.
Common Reagents and Conditions:
Oxidation: Common oxidizing agents include potassium permanganate and hydrogen peroxide.
Reduction: Sodium borohydride and lithium aluminum hydride are frequently used reducing agents.
Substitution: Halogens, nitrating agents, and sulfonating agents are commonly used for electrophilic substitution reactions.
Major Products: The major products formed from these reactions depend on the specific conditions and reagents used. For example, oxidation can yield quinones, while reduction can produce dihydrochalcones .
Vergleich Mit ähnlichen Verbindungen
3,2’-Dihydroxychalcone can be compared with other chalcones and flavonoids:
Eigenschaften
IUPAC Name |
(E)-1-(2-hydroxyphenyl)-3-(3-hydroxyphenyl)prop-2-en-1-one | |
---|---|---|
Source | PubChem | |
URL | https://pubchem.ncbi.nlm.nih.gov | |
Description | Data deposited in or computed by PubChem | |
InChI |
InChI=1S/C15H12O3/c16-12-5-3-4-11(10-12)8-9-15(18)13-6-1-2-7-14(13)17/h1-10,16-17H/b9-8+ | |
Source | PubChem | |
URL | https://pubchem.ncbi.nlm.nih.gov | |
Description | Data deposited in or computed by PubChem | |
InChI Key |
BLEVPIDFNNFTHJ-CMDGGOBGSA-N | |
Source | PubChem | |
URL | https://pubchem.ncbi.nlm.nih.gov | |
Description | Data deposited in or computed by PubChem | |
Canonical SMILES |
C1=CC=C(C(=C1)C(=O)C=CC2=CC(=CC=C2)O)O | |
Source | PubChem | |
URL | https://pubchem.ncbi.nlm.nih.gov | |
Description | Data deposited in or computed by PubChem | |
Isomeric SMILES |
C1=CC=C(C(=C1)C(=O)/C=C/C2=CC(=CC=C2)O)O | |
Source | PubChem | |
URL | https://pubchem.ncbi.nlm.nih.gov | |
Description | Data deposited in or computed by PubChem | |
Molecular Formula |
C15H12O3 | |
Source | PubChem | |
URL | https://pubchem.ncbi.nlm.nih.gov | |
Description | Data deposited in or computed by PubChem | |
DSSTOX Substance ID |
DTXSID601345756 | |
Record name | 2',3-Dihydroxychalcone | |
Source | EPA DSSTox | |
URL | https://comptox.epa.gov/dashboard/DTXSID601345756 | |
Description | DSSTox provides a high quality public chemistry resource for supporting improved predictive toxicology. | |
Molecular Weight |
240.25 g/mol | |
Source | PubChem | |
URL | https://pubchem.ncbi.nlm.nih.gov | |
Description | Data deposited in or computed by PubChem | |
CAS No. |
36574-83-1 | |
Record name | MLS002693861 | |
Source | DTP/NCI | |
URL | https://dtp.cancer.gov/dtpstandard/servlet/dwindex?searchtype=NSC&outputformat=html&searchlist=73257 | |
Description | The NCI Development Therapeutics Program (DTP) provides services and resources to the academic and private-sector research communities worldwide to facilitate the discovery and development of new cancer therapeutic agents. | |
Explanation | Unless otherwise indicated, all text within NCI products is free of copyright and may be reused without our permission. Credit the National Cancer Institute as the source. | |
Record name | 2',3-Dihydroxychalcone | |
Source | EPA DSSTox | |
URL | https://comptox.epa.gov/dashboard/DTXSID601345756 | |
Description | DSSTox provides a high quality public chemistry resource for supporting improved predictive toxicology. | |
Retrosynthesis Analysis
AI-Powered Synthesis Planning: Our tool employs the Template_relevance Pistachio, Template_relevance Bkms_metabolic, Template_relevance Pistachio_ringbreaker, Template_relevance Reaxys, Template_relevance Reaxys_biocatalysis model, leveraging a vast database of chemical reactions to predict feasible synthetic routes.
One-Step Synthesis Focus: Specifically designed for one-step synthesis, it provides concise and direct routes for your target compounds, streamlining the synthesis process.
Accurate Predictions: Utilizing the extensive PISTACHIO, BKMS_METABOLIC, PISTACHIO_RINGBREAKER, REAXYS, REAXYS_BIOCATALYSIS database, our tool offers high-accuracy predictions, reflecting the latest in chemical research and data.
Strategy Settings
Precursor scoring | Relevance Heuristic |
---|---|
Min. plausibility | 0.01 |
Model | Template_relevance |
Template Set | Pistachio/Bkms_metabolic/Pistachio_ringbreaker/Reaxys/Reaxys_biocatalysis |
Top-N result to add to graph | 6 |
Feasible Synthetic Routes
Q1: How does the presence of hydroxyl groups on the A-ring of 3,2'-dihydroxychalcone influence its glucuronidation by UGT enzymes?
A: Research indicates that the presence of a hydroxyl group on the A-ring of 3,2'-dihydroxychalcone can significantly impact its glucuronidation by specific UGT1A isoforms. For instance, when reacting with UGT1A9, the addition of a hydroxyl group to the A-ring of dihydroxychalcones leads to increased formation of the 2'-O-glucuronide product. [] This suggests that the A-ring hydroxyl group may enhance the interaction between the enzyme and the substrate at the 2' position, promoting glucuronidation at that site.
Q2: Does 3,2'-dihydroxychalcone undergo cyclization during glucuronidation by UGT enzymes, and if so, which enzyme exhibits this activity?
A: Yes, 3,2'-dihydroxychalcone can undergo cyclization to form its corresponding flavanone glucuronide during glucuronidation. Specifically, UGT1A7 has been shown to promote both the cyclization of 3,2'-dihydroxychalcone and the formation of its corresponding flavanone glucuronide. [] This highlights the diverse catalytic activities of different UGT1A isoforms and their ability to influence the metabolic fate of chalcones.
Haftungsausschluss und Informationen zu In-Vitro-Forschungsprodukten
Bitte beachten Sie, dass alle Artikel und Produktinformationen, die auf BenchChem präsentiert werden, ausschließlich zu Informationszwecken bestimmt sind. Die auf BenchChem zum Kauf angebotenen Produkte sind speziell für In-vitro-Studien konzipiert, die außerhalb lebender Organismen durchgeführt werden. In-vitro-Studien, abgeleitet von dem lateinischen Begriff "in Glas", beinhalten Experimente, die in kontrollierten Laborumgebungen unter Verwendung von Zellen oder Geweben durchgeführt werden. Es ist wichtig zu beachten, dass diese Produkte nicht als Arzneimittel oder Medikamente eingestuft sind und keine Zulassung der FDA für die Vorbeugung, Behandlung oder Heilung von medizinischen Zuständen, Beschwerden oder Krankheiten erhalten haben. Wir müssen betonen, dass jede Form der körperlichen Einführung dieser Produkte in Menschen oder Tiere gesetzlich strikt untersagt ist. Es ist unerlässlich, sich an diese Richtlinien zu halten, um die Einhaltung rechtlicher und ethischer Standards in Forschung und Experiment zu gewährleisten.