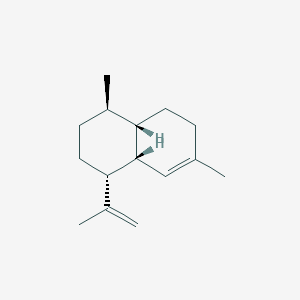
Amorphadiene
Übersicht
Beschreibung
Amorphadiene is a precursor to artemisinin . It is a sesquiterpene product synthesized by the cyclization of farnesyl pyrophosphate . The IUPAC name for Amorphadiene is 6α,7β,10α-Cadina-4,11-diene .
Synthesis Analysis
Amorphadiene synthase (ADS) is a key enzyme in the artemisinin biosynthetic pathway. ADS promotes the first step of artemisinin synthesis by cyclizing faresyl pyrophosphate to synthesize the sesquiterpene product amorpha-4,11-diene . In a study, it was found that HMG-CoA and acetyl-CoA supply are two limiting bottlenecks for amorphadiene production .Molecular Structure Analysis
The molecular structure of Amorphadiene is closely related to ADS genes found in the genome of Chrysanthemum nankingense . A catalytic model of ADS was suggested by the observation of TEAS, in which FDP binding placed Phe 525 next to Trp 271 to form an extended aromatic box, and the carbocation intermediates were stabilized by the nucleophilicity of the Trp 271 indole ring .Chemical Reactions Analysis
Amorphadiene is a byproduct from the fermentation process that can be obtained in very high titers (up to 120 g/ L), and can be converted into artemisinin . Quantum chemical studies on the formation of amorphadiene and the amorphene sesquiterpenes are described. These natural products are commonly thought to arise from distinct pathways involving initial 1,6- and 1,10-cyclization of farnesyl diphosphate, respectively .Physical And Chemical Properties Analysis
Amorphadiene has a chemical formula of C15H24 and a molar mass of 204.35 g/mol. Its density is 0.869 g/ml .Wissenschaftliche Forschungsanwendungen
Amorphadiene in Microbial Cell Factories
Amorphadiene is a key intermediate in the biosynthesis of artemisinin, which is a potent antimalarial drug. The compound has been the subject of extensive research to optimize its production through microbial engineering. One innovative approach involves the use of a co-culture system of Yarrowia lipolytica strains, which has shown significant improvements in amorphadiene production. This method leverages the subcellular localization of amorphadiene synthase into the endoplasmic reticulum and utilizes mixed carbon sources to enhance production .
Co-Culture Engineering for Enhanced Production
The interaction between two Yarrowia lipolytica strains, Po1g and Po1f, in a co-culture system represents a form of commensalism. The Po1g strain provides the substrate for the Po1f strain, aiding in the improved titer of amorphadiene. This modular co-culture engineering is a promising avenue for expanding the biosynthesis of amorphadiene and potentially other valuable compounds .
Optimization of Carbon Source Utilization
Research has also focused on optimizing the utilization of carbon sources in the medium to improve amorphadiene production. By co-utilizing acetate and xylose as secondary carbon sources along with glucose, researchers aim to divide labor between strains and enhance overall efficiency. This strategy could lead to more cost-effective and scalable production methods for amorphadiene and its derivatives .
Zukünftige Richtungen
Wirkmechanismus
Target of Action
Amorphadiene’s primary target is the biosynthetic pathway of artemisinin , a potent antimalarial drug . The key enzyme in this pathway is Amorpha-4,11-diene synthase (ADS) . ADS catalyzes the first committed step in the artemisinin biosynthetic pathway, which is the first catalytic reaction enzymatically and genetically characterized in artemisinin biosynthesis .
Mode of Action
Amorphadiene interacts with its target, ADS, through a process known as cyclization of farnesyl pyrophosphate (FPP) . This interaction results in the production of amorphadiene, a crucial precursor in the biosynthesis of artemisinin .
Biochemical Pathways
Amorphadiene is involved in the artemisinin biosynthetic pathway . This pathway begins with the conversion of acetyl-CoA to isopentenyl pyrophosphate (IPP) and its isomer dimethylallyl pyrophosphate (DMAPP) through the mevalonate pathway . These compounds are then converted to FPP by the action of the farnesyl pyrophosphate synthase (FPS) . ADS then catalyzes the cyclization of FPP to produce amorphadiene .
Pharmacokinetics
The production of amorphadiene and artemisinin from metabolically engineered microbes may provide an alternate to plant secondary metabolite extraction .
Result of Action
The result of amorphadiene’s action is the production of artemisinin , a potent antimalarial drug . Artemisinin and its derivatives demonstrate additional anticancer, anti-inflammation, antiviral, and anti-SARS-CoV-2 activity .
Action Environment
The action of amorphadiene is influenced by the metabolic environment of the host organism . For instance, in the oleaginous yeast Yarrowia lipolytica, the lipogenic acetyl-CoA pathways and the endogenous mevalonate pathway were harnessed for amorphadiene production . Additionally, the enlargement of the endoplasmic reticulum (ER) surface area caused by the deletion of the PAH1 gene provided more subcellular ER space for the action of the ADS-tagged gene, further increasing amorphadiene production .
Eigenschaften
IUPAC Name |
(1R,4R,4aS,8aR)-4,7-dimethyl-1-prop-1-en-2-yl-1,2,3,4,4a,5,6,8a-octahydronaphthalene | |
---|---|---|
Source | PubChem | |
URL | https://pubchem.ncbi.nlm.nih.gov | |
Description | Data deposited in or computed by PubChem | |
InChI |
InChI=1S/C15H24/c1-10(2)13-8-6-12(4)14-7-5-11(3)9-15(13)14/h9,12-15H,1,5-8H2,2-4H3/t12-,13+,14+,15-/m1/s1 | |
Source | PubChem | |
URL | https://pubchem.ncbi.nlm.nih.gov | |
Description | Data deposited in or computed by PubChem | |
InChI Key |
HMTAHNDPLDKYJT-CBBWQLFWSA-N | |
Source | PubChem | |
URL | https://pubchem.ncbi.nlm.nih.gov | |
Description | Data deposited in or computed by PubChem | |
Canonical SMILES |
CC1CCC(C2C1CCC(=C2)C)C(=C)C | |
Source | PubChem | |
URL | https://pubchem.ncbi.nlm.nih.gov | |
Description | Data deposited in or computed by PubChem | |
Isomeric SMILES |
C[C@@H]1CC[C@H]([C@@H]2[C@H]1CCC(=C2)C)C(=C)C | |
Source | PubChem | |
URL | https://pubchem.ncbi.nlm.nih.gov | |
Description | Data deposited in or computed by PubChem | |
Molecular Formula |
C15H24 | |
Source | PubChem | |
URL | https://pubchem.ncbi.nlm.nih.gov | |
Description | Data deposited in or computed by PubChem | |
DSSTOX Substance ID |
DTXSID50239106 | |
Record name | Amorphadiene | |
Source | EPA DSSTox | |
URL | https://comptox.epa.gov/dashboard/DTXSID50239106 | |
Description | DSSTox provides a high quality public chemistry resource for supporting improved predictive toxicology. | |
Molecular Weight |
204.35 g/mol | |
Source | PubChem | |
URL | https://pubchem.ncbi.nlm.nih.gov | |
Description | Data deposited in or computed by PubChem | |
CAS RN |
92692-39-2 | |
Record name | (-)-Amorpha-4,11-diene | |
Source | CAS Common Chemistry | |
URL | https://commonchemistry.cas.org/detail?cas_rn=92692-39-2 | |
Description | CAS Common Chemistry is an open community resource for accessing chemical information. Nearly 500,000 chemical substances from CAS REGISTRY cover areas of community interest, including common and frequently regulated chemicals, and those relevant to high school and undergraduate chemistry classes. This chemical information, curated by our expert scientists, is provided in alignment with our mission as a division of the American Chemical Society. | |
Explanation | The data from CAS Common Chemistry is provided under a CC-BY-NC 4.0 license, unless otherwise stated. | |
Record name | Amorphadiene | |
Source | ChemIDplus | |
URL | https://pubchem.ncbi.nlm.nih.gov/substance/?source=chemidplus&sourceid=0092692392 | |
Description | ChemIDplus is a free, web search system that provides access to the structure and nomenclature authority files used for the identification of chemical substances cited in National Library of Medicine (NLM) databases, including the TOXNET system. | |
Record name | Amorphadiene | |
Source | EPA DSSTox | |
URL | https://comptox.epa.gov/dashboard/DTXSID50239106 | |
Description | DSSTox provides a high quality public chemistry resource for supporting improved predictive toxicology. | |
Retrosynthesis Analysis
AI-Powered Synthesis Planning: Our tool employs the Template_relevance Pistachio, Template_relevance Bkms_metabolic, Template_relevance Pistachio_ringbreaker, Template_relevance Reaxys, Template_relevance Reaxys_biocatalysis model, leveraging a vast database of chemical reactions to predict feasible synthetic routes.
One-Step Synthesis Focus: Specifically designed for one-step synthesis, it provides concise and direct routes for your target compounds, streamlining the synthesis process.
Accurate Predictions: Utilizing the extensive PISTACHIO, BKMS_METABOLIC, PISTACHIO_RINGBREAKER, REAXYS, REAXYS_BIOCATALYSIS database, our tool offers high-accuracy predictions, reflecting the latest in chemical research and data.
Strategy Settings
Precursor scoring | Relevance Heuristic |
---|---|
Min. plausibility | 0.01 |
Model | Template_relevance |
Template Set | Pistachio/Bkms_metabolic/Pistachio_ringbreaker/Reaxys/Reaxys_biocatalysis |
Top-N result to add to graph | 6 |
Feasible Synthetic Routes
Haftungsausschluss und Informationen zu In-Vitro-Forschungsprodukten
Bitte beachten Sie, dass alle Artikel und Produktinformationen, die auf BenchChem präsentiert werden, ausschließlich zu Informationszwecken bestimmt sind. Die auf BenchChem zum Kauf angebotenen Produkte sind speziell für In-vitro-Studien konzipiert, die außerhalb lebender Organismen durchgeführt werden. In-vitro-Studien, abgeleitet von dem lateinischen Begriff "in Glas", beinhalten Experimente, die in kontrollierten Laborumgebungen unter Verwendung von Zellen oder Geweben durchgeführt werden. Es ist wichtig zu beachten, dass diese Produkte nicht als Arzneimittel oder Medikamente eingestuft sind und keine Zulassung der FDA für die Vorbeugung, Behandlung oder Heilung von medizinischen Zuständen, Beschwerden oder Krankheiten erhalten haben. Wir müssen betonen, dass jede Form der körperlichen Einführung dieser Produkte in Menschen oder Tiere gesetzlich strikt untersagt ist. Es ist unerlässlich, sich an diese Richtlinien zu halten, um die Einhaltung rechtlicher und ethischer Standards in Forschung und Experiment zu gewährleisten.