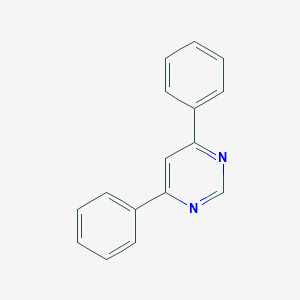
4,6-Diphenylpyrimidine
Übersicht
Beschreibung
4,6-Diphenylpyrimidine is a heterocyclic aromatic organic compound characterized by a pyrimidine ring substituted with phenyl groups at the 4 and 6 positions. This compound is of significant interest due to its diverse applications in medicinal chemistry, particularly in the development of anticancer agents and other therapeutic drugs.
Wissenschaftliche Forschungsanwendungen
4,6-Diphenylpyrimidine has a wide range of applications in scientific research:
Chemistry: Used as a building block in the synthesis of more complex organic molecules.
Biology: Studied for its interactions with biological macromolecules and potential as a biochemical probe.
Medicine: Investigated for its anticancer properties, particularly as an inhibitor of Aurora kinase A, which plays a crucial role in cell division.
Wirkmechanismus
Target of Action
4,6-Diphenylpyrimidine primarily targets Histone Deacetylases (HDACs) and Monoamine Oxidase (MAO) and Acetylcholinesterase enzymes (AChE) . HDACs are a class of enzymes that remove acetyl groups from an ε-N-acetyl lysine amino acid on a histone, allowing the histones to wrap the DNA more tightly. MAO and AChE are enzymes that play a crucial role in neurotransmission, and their inhibition can have significant effects on mood and cognition.
Mode of Action
This compound interacts with its targets by inhibiting their activity. It selectively inhibits the activity of Aurora kinase A (AURKA) , a key regulator of mitosis. It also inhibits the activity of HDACs , which can lead to changes in gene expression. Furthermore, it inhibits MAO-A and AChE enzymes , which can affect neurotransmission.
Biochemical Pathways
The inhibition of AURKA by this compound affects the cell cycle , particularly the transition from G2 to M phase . The inhibition of HDACs can affect numerous biochemical pathways due to the broad impact on gene expression . The inhibition of MAO-A and AChE can affect the metabolism of various neurotransmitters, potentially impacting mood and cognition .
Pharmacokinetics
It is known that the compound has been optimized for better efficacy, physicochemical, and adme properties .
Result of Action
The inhibition of AURKA by this compound can lead to cell cycle arrest at the G2/M phase and induce caspase-mediated apoptotic cell death . The inhibition of HDACs can lead to changes in gene expression, potentially affecting a wide range of cellular processes . The inhibition of MAO-A and AChE can affect neurotransmission, potentially impacting mood and cognition .
Safety and Hazards
The safety information for 4,6-Diphenylpyrimidine indicates that it is harmful if swallowed and causes serious eye irritation . Precautionary measures include avoiding dust formation, avoiding breathing mist, gas or vapours, avoiding contact with skin and eye, and using personal protective equipment .
Zukünftige Richtungen
Biochemische Analyse
Biochemical Properties
4,6-Diphenylpyrimidine has been found to interact with various enzymes and proteins. For instance, it has been reported to inhibit Aurora kinase A, an enzyme involved in cell cycle regulation . It also shows interactions with monoamine oxidase and acetylcholinesterase, enzymes that are potential targets for the treatment of Alzheimer’s disease .
Cellular Effects
In cellular assays, this compound derivatives have shown promising antiproliferative activities against different tumor cell lines . They have been found to influence cell function by affecting cell signaling pathways, gene expression, and cellular metabolism .
Molecular Mechanism
This compound exerts its effects at the molecular level through various mechanisms. For instance, it has been found to inhibit Aurora kinase A activity and reduce phosphorylation of Aurora kinase A at Thr283 in HCT116 human colon cancer cells . This suggests that this compound may exert its effects through enzyme inhibition or activation, and changes in gene expression .
Temporal Effects in Laboratory Settings
Its derivatives have shown promising results in long-term survival assays, suggesting potential stability and long-term effects on cellular function .
Metabolic Pathways
As a pyrimidine derivative, it may be involved in pyrimidine metabolism, which includes a series of biochemical reactions involving enzymes and cofactors .
Vorbereitungsmethoden
Synthetic Routes and Reaction Conditions: The synthesis of 4,6-Diphenylpyrimidine typically involves the condensation of benzaldehyde with acetophenone in the presence of a base, followed by cyclization with urea or guanidine. One efficient method is the Biginelli-like reaction, which involves the reaction of aromatic aldehydes, 1,2-diphenylethanone, and urea or guanidine carbonate under solvent-free conditions . This method is advantageous due to its mild reaction conditions, short reaction time, and environmental friendliness.
Industrial Production Methods: Industrial production of this compound may involve similar synthetic routes but on a larger scale, utilizing continuous flow reactors to ensure consistent product quality and yield. The use of catalysts and optimized reaction conditions can further enhance the efficiency of the production process.
Analyse Chemischer Reaktionen
Types of Reactions: 4,6-Diphenylpyrimidine undergoes various chemical reactions, including:
Oxidation: The compound can be oxidized to form corresponding pyrimidine oxides.
Reduction: Reduction reactions can yield dihydropyrimidine derivatives.
Substitution: Electrophilic and nucleophilic substitution reactions can introduce different functional groups onto the pyrimidine ring.
Common Reagents and Conditions:
Oxidation: Common oxidizing agents include hydrogen peroxide and potassium permanganate.
Reduction: Reducing agents such as sodium borohydride and lithium aluminum hydride are often used.
Substitution: Reagents like halogens, alkyl halides, and organometallic compounds are employed under various conditions.
Major Products:
Oxidation: Pyrimidine oxides.
Reduction: Dihydropyrimidine derivatives.
Substitution: Various substituted pyrimidine derivatives depending on the reagents used.
Vergleich Mit ähnlichen Verbindungen
4,6-Diphenylpyrimidine can be compared with other pyrimidine derivatives such as:
2,4,6-Trisubstituted Pyrimidine: Similar in structure but with different substituents, leading to varied chemical and physical properties.
This compound Derivatives: These include compounds with additional functional groups that enhance their biological activity, such as dual inhibitors of monoamine oxidase and acetylcholinesterase for Alzheimer’s disease treatment.
Uniqueness: this compound is unique due to its dual role in medicinal chemistry as both an anticancer agent and a potential treatment for neurodegenerative diseases. Its ability to undergo various chemical reactions also makes it a versatile compound for synthetic applications.
Eigenschaften
IUPAC Name |
4,6-diphenylpyrimidine | |
---|---|---|
Source | PubChem | |
URL | https://pubchem.ncbi.nlm.nih.gov | |
Description | Data deposited in or computed by PubChem | |
InChI |
InChI=1S/C16H12N2/c1-3-7-13(8-4-1)15-11-16(18-12-17-15)14-9-5-2-6-10-14/h1-12H | |
Source | PubChem | |
URL | https://pubchem.ncbi.nlm.nih.gov | |
Description | Data deposited in or computed by PubChem | |
InChI Key |
BWRMZQGIDWILAU-UHFFFAOYSA-N | |
Source | PubChem | |
URL | https://pubchem.ncbi.nlm.nih.gov | |
Description | Data deposited in or computed by PubChem | |
Canonical SMILES |
C1=CC=C(C=C1)C2=CC(=NC=N2)C3=CC=CC=C3 | |
Source | PubChem | |
URL | https://pubchem.ncbi.nlm.nih.gov | |
Description | Data deposited in or computed by PubChem | |
Molecular Formula |
C16H12N2 | |
Source | PubChem | |
URL | https://pubchem.ncbi.nlm.nih.gov | |
Description | Data deposited in or computed by PubChem | |
DSSTOX Substance ID |
DTXSID70355817 | |
Record name | 4,6-diphenylpyrimidine | |
Source | EPA DSSTox | |
URL | https://comptox.epa.gov/dashboard/DTXSID70355817 | |
Description | DSSTox provides a high quality public chemistry resource for supporting improved predictive toxicology. | |
Molecular Weight |
232.28 g/mol | |
Source | PubChem | |
URL | https://pubchem.ncbi.nlm.nih.gov | |
Description | Data deposited in or computed by PubChem | |
Solubility |
4.6 [ug/mL] (The mean of the results at pH 7.4) | |
Record name | SID47195966 | |
Source | Burnham Center for Chemical Genomics | |
URL | https://pubchem.ncbi.nlm.nih.gov/bioassay/1996#section=Data-Table | |
Description | Aqueous solubility in buffer at pH 7.4 | |
CAS No. |
3977-48-8 | |
Record name | 4,6-diphenylpyrimidine | |
Source | EPA DSSTox | |
URL | https://comptox.epa.gov/dashboard/DTXSID70355817 | |
Description | DSSTox provides a high quality public chemistry resource for supporting improved predictive toxicology. | |
Synthesis routes and methods
Procedure details
Retrosynthesis Analysis
AI-Powered Synthesis Planning: Our tool employs the Template_relevance Pistachio, Template_relevance Bkms_metabolic, Template_relevance Pistachio_ringbreaker, Template_relevance Reaxys, Template_relevance Reaxys_biocatalysis model, leveraging a vast database of chemical reactions to predict feasible synthetic routes.
One-Step Synthesis Focus: Specifically designed for one-step synthesis, it provides concise and direct routes for your target compounds, streamlining the synthesis process.
Accurate Predictions: Utilizing the extensive PISTACHIO, BKMS_METABOLIC, PISTACHIO_RINGBREAKER, REAXYS, REAXYS_BIOCATALYSIS database, our tool offers high-accuracy predictions, reflecting the latest in chemical research and data.
Strategy Settings
Precursor scoring | Relevance Heuristic |
---|---|
Min. plausibility | 0.01 |
Model | Template_relevance |
Template Set | Pistachio/Bkms_metabolic/Pistachio_ringbreaker/Reaxys/Reaxys_biocatalysis |
Top-N result to add to graph | 6 |
Feasible Synthetic Routes
Q1: How do 4,6-diphenylpyrimidine derivatives interact with Monoamine Oxidase A (MAO-A) and Acetylcholinesterase (AChE)?
A1: this compound derivatives act as dual inhibitors of MAO-A and AChE. [] These compounds bind to the hydrophobic cavities of both enzymes. Molecular dynamics simulations demonstrate the stability of this binding interaction over time. [] By inhibiting MAO-A, these derivatives prevent the breakdown of neurotransmitters like dopamine, potentially offering neuroprotective effects. [] Inhibition of AChE, on the other hand, leads to increased levels of acetylcholine, another crucial neurotransmitter involved in learning and memory. []
Q2: Do this compound derivatives exhibit neuroprotective properties?
A2: Yes, studies show that these derivatives can protect neuronal cells from damage induced by 6-hydroxydopamine (6-OHDA) and hydrogen peroxide (H2O2), both of which are implicated in neurodegenerative processes. [] This neuroprotective effect is attributed to their ability to inhibit MAO-A and AChE, as well as their antioxidant and metal-chelating properties. []
Q3: What is the core structure of this compound?
A3: As the name suggests, this compound consists of a pyrimidine ring with two phenyl groups attached at the 4th and 6th positions. This structure serves as a foundation for various modifications, leading to a diverse range of derivatives.
Q4: How can the structure of this compound be modified to create diverse derivatives?
A4: Several modifications are possible, including substitutions on the phenyl rings, introduction of various functional groups at different positions on the pyrimidine core, and alterations to the linker units when connecting the pyrimidine core to other pharmacophores. []
Q5: Do this compound derivatives exhibit any notable catalytic properties?
A5: While not extensively explored as catalysts, their structural features, particularly the presence of nitrogen atoms in the pyrimidine ring, suggest potential for coordination chemistry and catalytic applications. Further research is needed to fully understand their catalytic potential.
Q6: Have computational methods been employed in the study of this compound derivatives?
A6: Yes, molecular docking studies have been employed to predict the binding mode and affinity of these derivatives to target enzymes such as MAO-A and AChE. [] Molecular dynamics simulations have provided insights into the stability of these interactions over time, offering valuable information for further drug design efforts. []
Q7: How do structural modifications to this compound influence its biological activity?
A7: Studies have shown that incorporating propargyl groups into this compound enhances its inhibitory activity against both MAO-A and AChE. [] Furthermore, the position and nature of substituents on the phenyl rings significantly impact the potency and selectivity towards these enzymes. [] For instance, compound VB1, featuring specific substitutions, emerged as a potent dual inhibitor of MAO-A and butyrylcholinesterase (BuChE). [] On the other hand, compound VB8 displayed remarkable potency towards AChE. []
Q8: Have any specific formulation strategies been investigated to improve the stability, solubility, or bioavailability of this compound derivatives?
A8: While the provided research papers do not delve into specific formulation strategies, the development of suitable formulations will be crucial for translating these compounds into viable therapeutic agents. Strategies like nanoparticle encapsulation, inclusion complexes, and prodrug approaches could be explored to enhance their pharmacological properties.
Q9: What in vitro models have been used to evaluate the efficacy of this compound derivatives?
A9: Researchers have employed human neuroblastoma SH-SY5Y cells as an in vitro model to assess the neuroprotective effects of these compounds against induced toxicity. [] These cells serve as a valuable tool to study the molecular mechanisms underlying the observed neuroprotection.
Haftungsausschluss und Informationen zu In-Vitro-Forschungsprodukten
Bitte beachten Sie, dass alle Artikel und Produktinformationen, die auf BenchChem präsentiert werden, ausschließlich zu Informationszwecken bestimmt sind. Die auf BenchChem zum Kauf angebotenen Produkte sind speziell für In-vitro-Studien konzipiert, die außerhalb lebender Organismen durchgeführt werden. In-vitro-Studien, abgeleitet von dem lateinischen Begriff "in Glas", beinhalten Experimente, die in kontrollierten Laborumgebungen unter Verwendung von Zellen oder Geweben durchgeführt werden. Es ist wichtig zu beachten, dass diese Produkte nicht als Arzneimittel oder Medikamente eingestuft sind und keine Zulassung der FDA für die Vorbeugung, Behandlung oder Heilung von medizinischen Zuständen, Beschwerden oder Krankheiten erhalten haben. Wir müssen betonen, dass jede Form der körperlichen Einführung dieser Produkte in Menschen oder Tiere gesetzlich strikt untersagt ist. Es ist unerlässlich, sich an diese Richtlinien zu halten, um die Einhaltung rechtlicher und ethischer Standards in Forschung und Experiment zu gewährleisten.