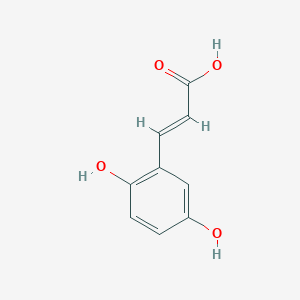
2,5-Dihydroxyzimtsäure
Übersicht
Beschreibung
2,5-Dihydroxycinnamic acid is a hydroxycinnamic acid and an isomer of caffeic acid . It is a cinnamate ester, a member of hydroquinones, and a methyl ester .
Synthesis Analysis
2,5-Dihydroxycinnamic acid is produced by Elbs persulfate oxidation of o-Coumaric acid .
Molecular Structure Analysis
The molecular formula of 2,5-Dihydroxycinnamic acid is C9H8O4, and its molar mass is 180.159 g·mol −1 . For more detailed molecular structure analysis, you may refer to resources like SpectraBase .
Chemical Reactions Analysis
Hydroxycinnamic acids, including 2,5-Dihydroxycinnamic acid, are known to be potent antioxidants. Their antioxidant activity is related to their hydrogen or electron donating capacity and the ability to delocalize/stabilize the resulting phenoxyl radical within their structure .
Physical and Chemical Properties Analysis
The physical and chemical properties of 2,5-Dihydroxycinnamic acid include its molecular weight of 180.16 g/mol . For more detailed physical and chemical properties, you may refer to resources like PubChem .
Wissenschaftliche Forschungsanwendungen
Hautpflege und -störungen
2,5-Dihydroxyzimtsäure wurde, wie andere Hydroxyzimtsäuren, auf ihr Potenzial zur Behandlung von Hauterkrankungen untersucht . Diese Verbindungen haben antioxidative, entzündungshemmende und antibakterielle Wirkungen gezeigt , was macht sie potenziell nützlich bei der Behandlung und Wiederherstellung des Gleichgewichts von Hautkrankheiten und -erkrankungen auf verschiedenen Ebenen .
Antioxidative Eigenschaften
Hydroxyzimtsäuren, einschließlich this compound, sind für ihre antioxidativen Eigenschaften bekannt . Sie können den Abbau einiger pflanzlicher und Lebensmittelbestandteile wie Anthocyanine schützen .
Entzündungshemmende Eigenschaften
Die entzündungshemmenden Eigenschaften von Hydroxyzimtsäuren können bei Erkrankungen hilfreich sein, die durch Entzündungen gekennzeichnet sind . Dies umfasst verschiedene Hauterkrankungen sowie möglicherweise auch andere entzündliche Erkrankungen .
Antibakterielle Eigenschaften
Hydroxyzimtsäuren haben antibakterielle Wirkungen gezeigt . Dies könnte sie in Situationen nützlich machen, in denen bakterielle Infektionen ein Problem darstellen, z. B. bei der Wundversorgung .
Wechselwirkung mit Lebensmittel-Biomakromolekülen
Hydroxyzimtsäuren, einschließlich this compound, können mit Lebensmittel-Biomakromolekülen wie Proteinen und Oligo- und Polysacchariden interagieren
Wirkmechanismus
Target of Action
2,5-Dihydroxycinnamic acid, a hydroxycinnamic acid derivative , is known to interact with receptor protein-tyrosine kinase (EC 2.7.10.1) . This enzyme plays a crucial role in many cellular processes, including cell growth and differentiation, metabolism, and cell cycle control.
Mode of Action
The compound acts as an inhibitor of receptor protein-tyrosine kinase . By binding to this enzyme, it interferes with its activity, leading to changes in the cellular processes controlled by this enzyme.
Result of Action
The molecular and cellular effects of 2,5-Dihydroxycinnamic acid’s action are largely dependent on its interaction with receptor protein-tyrosine kinase . By inhibiting this enzyme, it can potentially affect cell growth, differentiation, and metabolism.
Safety and Hazards
Zukünftige Richtungen
Hydroxycinnamic acids and their derivatives, including 2,5-Dihydroxycinnamic acid, have been investigated for their anti-oxidant, anti-inflammatory, and anti-bacterial activities. They have potential roles as valid alternatives to current therapies for managing and rebalancing skin disorders and diseases at different levels . The use of cinnamic acid, including 2,5-Dihydroxycinnamic acid, provides an approach to the research and development of biobased plastics for the reduction of global warming caused by the increasing amounts of carbon dioxide .
Biochemische Analyse
Biochemical Properties
They are involved in the shikimate pathway, which is responsible for the biosynthesis of aromatic amino acids and phenylpropanoids .
Cellular Effects
Natural compounds, including hydroxycinnamic acids, have been suggested to regulate glucose-lipid metabolism by influencing short-chain fatty acids (SCFAs) . This metabolism is key to maintaining energy balance and normal physiological functions in the body .
Molecular Mechanism
Hydroxycinnamic acids are known to exert their effects through various mechanisms, including antioxidant, anti-inflammatory, and antibacterial activities .
Temporal Effects in Laboratory Settings
It is known that hydroxycinnamic acids and their derivatives have been investigated for their antioxidant, anti-inflammatory, and antibacterial activities .
Metabolic Pathways
2,5-Dihydroxycinnamic acid is involved in the shikimate pathway, which is responsible for the biosynthesis of aromatic amino acids and phenylpropanoids . This pathway is crucial for plant metabolism and defense .
Transport and Distribution
It is known that hydroxycinnamic acids can be transported and distributed within cells and tissues through various mechanisms .
Subcellular Localization
The prediction of protein subcellular localization from their amino acid sequences has a long history in bioinformatics and is still actively developing .
Eigenschaften
IUPAC Name |
(E)-3-(2,5-dihydroxyphenyl)prop-2-enoic acid | |
---|---|---|
Source | PubChem | |
URL | https://pubchem.ncbi.nlm.nih.gov | |
Description | Data deposited in or computed by PubChem | |
InChI |
InChI=1S/C9H8O4/c10-7-2-3-8(11)6(5-7)1-4-9(12)13/h1-5,10-11H,(H,12,13)/b4-1+ | |
Source | PubChem | |
URL | https://pubchem.ncbi.nlm.nih.gov | |
Description | Data deposited in or computed by PubChem | |
InChI Key |
JXIPYOZBOMUUCA-DAFODLJHSA-N | |
Source | PubChem | |
URL | https://pubchem.ncbi.nlm.nih.gov | |
Description | Data deposited in or computed by PubChem | |
Canonical SMILES |
C1=CC(=C(C=C1O)C=CC(=O)O)O | |
Source | PubChem | |
URL | https://pubchem.ncbi.nlm.nih.gov | |
Description | Data deposited in or computed by PubChem | |
Isomeric SMILES |
C1=CC(=C(C=C1O)/C=C/C(=O)O)O | |
Source | PubChem | |
URL | https://pubchem.ncbi.nlm.nih.gov | |
Description | Data deposited in or computed by PubChem | |
Molecular Formula |
C9H8O4 | |
Source | PubChem | |
URL | https://pubchem.ncbi.nlm.nih.gov | |
Description | Data deposited in or computed by PubChem | |
DSSTOX Substance ID |
DTXSID001030505 | |
Record name | 2,5-Dihydroxycinnamic acid | |
Source | EPA DSSTox | |
URL | https://comptox.epa.gov/dashboard/DTXSID001030505 | |
Description | DSSTox provides a high quality public chemistry resource for supporting improved predictive toxicology. | |
Molecular Weight |
180.16 g/mol | |
Source | PubChem | |
URL | https://pubchem.ncbi.nlm.nih.gov | |
Description | Data deposited in or computed by PubChem | |
CAS No. |
38489-67-7, 636-01-1 | |
Record name | 2,5-Dihydroxycinnamic acid | |
Source | ChemIDplus | |
URL | https://pubchem.ncbi.nlm.nih.gov/substance/?source=chemidplus&sourceid=0038489677 | |
Description | ChemIDplus is a free, web search system that provides access to the structure and nomenclature authority files used for the identification of chemical substances cited in National Library of Medicine (NLM) databases, including the TOXNET system. | |
Record name | 2,5-Dihydroxycinnamic acid | |
Source | EPA DSSTox | |
URL | https://comptox.epa.gov/dashboard/DTXSID001030505 | |
Description | DSSTox provides a high quality public chemistry resource for supporting improved predictive toxicology. | |
Record name | 2,5-DIHYDROXYCINNAMIC ACID | |
Source | FDA Global Substance Registration System (GSRS) | |
URL | https://gsrs.ncats.nih.gov/ginas/app/beta/substances/6EHK92QE99 | |
Description | The FDA Global Substance Registration System (GSRS) enables the efficient and accurate exchange of information on what substances are in regulated products. Instead of relying on names, which vary across regulatory domains, countries, and regions, the GSRS knowledge base makes it possible for substances to be defined by standardized, scientific descriptions. | |
Explanation | Unless otherwise noted, the contents of the FDA website (www.fda.gov), both text and graphics, are not copyrighted. They are in the public domain and may be republished, reprinted and otherwise used freely by anyone without the need to obtain permission from FDA. Credit to the U.S. Food and Drug Administration as the source is appreciated but not required. | |
Retrosynthesis Analysis
AI-Powered Synthesis Planning: Our tool employs the Template_relevance Pistachio, Template_relevance Bkms_metabolic, Template_relevance Pistachio_ringbreaker, Template_relevance Reaxys, Template_relevance Reaxys_biocatalysis model, leveraging a vast database of chemical reactions to predict feasible synthetic routes.
One-Step Synthesis Focus: Specifically designed for one-step synthesis, it provides concise and direct routes for your target compounds, streamlining the synthesis process.
Accurate Predictions: Utilizing the extensive PISTACHIO, BKMS_METABOLIC, PISTACHIO_RINGBREAKER, REAXYS, REAXYS_BIOCATALYSIS database, our tool offers high-accuracy predictions, reflecting the latest in chemical research and data.
Strategy Settings
Precursor scoring | Relevance Heuristic |
---|---|
Min. plausibility | 0.01 |
Model | Template_relevance |
Template Set | Pistachio/Bkms_metabolic/Pistachio_ringbreaker/Reaxys/Reaxys_biocatalysis |
Top-N result to add to graph | 6 |
Feasible Synthetic Routes
Q1: What is the structure of 2,5-Dihydroxycinnamic acid and what are its key spectroscopic characteristics?
A1: 2,5-Dihydroxycinnamic acid is a phenolic compound with the molecular formula C₉H₈O₄ and a molecular weight of 180.16 g/mol. It features a cinnamic acid backbone with two hydroxyl groups attached to the benzene ring at positions 2 and 5. Spectroscopically, it can be characterized by UV, IR, and NMR techniques. For example, [] characterized the compound using spectral methods, likely including NMR.
Q2: What are some natural sources of 2,5-Dihydroxycinnamic acid?
A2: This compound has been isolated from various plant sources. One notable example is Grevillea robusta, commonly known as the southern silky oak or silk oak. Researchers have identified 2,5-Dihydroxycinnamic acid and its esters with arbutin and glucose in the leaves of this plant. [, ] Additionally, it has been found in the underground parts of Isodon phyllostachys [], the flower buds of Tussilago farfara [], and Artemisia sacrorum Ledeb [].
Q3: What are the potential applications of 2,5-Dihydroxycinnamic acid in cancer research?
A4: Research suggests that 2,5-Dihydroxycinnamic acid derivatives could have anti-cancer properties. A study investigated a novel 2,5-Dihydroxycinnamic acid-based 5-lipoxygenase inhibitor and its effects on RCC4 renal cancer cells. The study revealed that this inhibitor induced apoptosis and potentially disrupted the autophagic flux in these cells. [] While promising, more research is needed to determine its therapeutic potential.
Q4: How has 2,5-Dihydroxycinnamic acid been used in studies on matrix-assisted laser desorption ionization (MALDI)?
A5: 2,5-Dihydroxycinnamic acid (DHB) has been used as a matrix in MALDI mass spectrometry. It has been shown to transfer less internal energy to analyte ions compared to other common matrices like CHCA and SA, particularly with picosecond laser excitation. This property makes DHB suitable for analyzing fragile biomolecules. []
Q5: Are there any known structure-activity relationships for 2,5-Dihydroxycinnamic acid derivatives?
A6: Yes, studies have explored the structure-activity relationship of 2,5-Dihydroxycinnamic acid derivatives, particularly in the context of antimyeloma activity. For example, research on caffeic acid phenethyl ester (CAPE) analogs, including the 2,5-dihydroxycinnamic acid 3-phenylpropyl ester (17), demonstrated potent anti-myeloma effects. These compounds were linked to the downregulation of Sp1 and the IKZF1-IRF4-MYC axis, revealing a potential mechanism of action. []
Q6: Has 2,5-Dihydroxycinnamic acid been investigated in relation to protein tyrosine kinases (PTKs)?
A7: Yes, research suggests a possible link between 2,5-Dihydroxycinnamic acid and PTK activity. One study explored the effects of 2,5-dihydroxycinnamic acid methyl ester, a PTK inhibitor, on the purinergic contraction of the guinea pig vas deferens. The study found that this compound inhibited the facilitation of purinergic contraction induced by sodium orthovanadate, a compound known to increase protein tyrosine phosphorylation. [] This suggests a potential role of 2,5-Dihydroxycinnamic acid or its derivatives in modulating PTK activity and downstream signaling pathways.
Haftungsausschluss und Informationen zu In-Vitro-Forschungsprodukten
Bitte beachten Sie, dass alle Artikel und Produktinformationen, die auf BenchChem präsentiert werden, ausschließlich zu Informationszwecken bestimmt sind. Die auf BenchChem zum Kauf angebotenen Produkte sind speziell für In-vitro-Studien konzipiert, die außerhalb lebender Organismen durchgeführt werden. In-vitro-Studien, abgeleitet von dem lateinischen Begriff "in Glas", beinhalten Experimente, die in kontrollierten Laborumgebungen unter Verwendung von Zellen oder Geweben durchgeführt werden. Es ist wichtig zu beachten, dass diese Produkte nicht als Arzneimittel oder Medikamente eingestuft sind und keine Zulassung der FDA für die Vorbeugung, Behandlung oder Heilung von medizinischen Zuständen, Beschwerden oder Krankheiten erhalten haben. Wir müssen betonen, dass jede Form der körperlichen Einführung dieser Produkte in Menschen oder Tiere gesetzlich strikt untersagt ist. Es ist unerlässlich, sich an diese Richtlinien zu halten, um die Einhaltung rechtlicher und ethischer Standards in Forschung und Experiment zu gewährleisten.