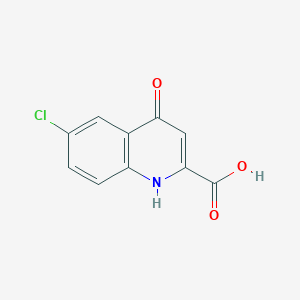
6-Chloro-4-oxo-1,4-dihydroquinoline-2-carboxylic acid
Übersicht
Beschreibung
6-Chlorokynurensäure ist eine Chinolinmonocarbonsäure, die strukturell durch ein Chloratom an Position 6 und eine Hydroxygruppe an Position 4 gekennzeichnet ist. Es ist ein potenter Antagonist des N-Methyl-D-Aspartat (NMDA)-Rezeptors, der speziell auf die Glycin-Bindungsstelle des Rezeptors abzielt . Diese Verbindung hat aufgrund ihrer potenziellen therapeutischen Anwendungen, insbesondere in der Neuroprotektion und bei antidepressiven Wirkungen, erhebliches Interesse geweckt .
Herstellungsmethoden
Die Synthese von 6-Chlorokynurensäure umfasst in der Regel mehrere Schritte:
Cyclisierungsreaktion: Ausgehend von kostengünstiger DL-Äpfelsäure wird eine Cyclisierungsreaktion durchgeführt, um eine Vorläuferverbindung zu bilden.
Ammonifizierungsreaktion: Der Vorläufer unterliegt einer Ammonifizierungsreaktion, um 6-Hydroxynicotinsäure zu ergeben.
Chlorierungsreaktion: Schließlich wird 6-Hydroxynicotinsäure einer Chlorierungsreaktion unterzogen, um 6-Chlorokynurensäure zu produzieren
Diese Reaktionen werden unter relativ einfachen Bedingungen durchgeführt, mit minimalen Umweltauswirkungen und hoher Ausbeute, was das Verfahren wirtschaftlich für die industrielle Produktion macht .
Chemische Reaktionsanalyse
6-Chlorokynurensäure unterliegt verschiedenen Arten von chemischen Reaktionen:
Oxidation: Sie kann oxidiert werden, um verschiedene Chinolinsäurederivate zu bilden.
Reduktion: Reduktionsreaktionen können die Chinolinringstruktur modifizieren.
Substitution: Das Chloratom an Position 6 kann unter geeigneten Bedingungen durch andere funktionelle Gruppen substituiert werden.
Häufig verwendete Reagenzien in diesen Reaktionen umfassen Oxidationsmittel wie hypochlorige Säure und Reduktionsmittel wie Natriumborhydrid . Die Hauptprodukte, die aus diesen Reaktionen gebildet werden, sind in der Regel andere Chinolinsäurederivate mit modifizierten funktionellen Gruppen .
Wissenschaftliche Forschungsanwendungen
6-Chlorokynurensäure hat eine breite Palette von wissenschaftlichen Forschungsanwendungen:
Neuroprotektion: Es wirkt als neuroprotektives Mittel, indem es den NMDA-Rezeptor antagonisiert und die durch Exzitotoxizität verursachte Neurodegeneration verhindert.
Antidepressive Wirkungen: Es wurde gezeigt, dass es schnelle antidepressive Wirkungen hervorruft, indem es die Expression von hippocampalen Micro-RNAs reguliert, die an den TrkB-ERK/Akt-Signalwegen beteiligt sind.
Glutamat-Wiederaufnahmehemmung: Es hemmt die Wiederaufnahme von Glutamat in synaptische Vesikel und moduliert so die synaptische Übertragung.
Wirkmechanismus
6-Chlorokynurensäure übt seine Wirkungen hauptsächlich durch Antagonismus des NMDA-Rezeptors an der Glycin-Bindungsstelle aus. Diese Hemmung verhindert, dass der exzitatorische Neurotransmitter Glutamat an den Rezeptor bindet, wodurch die neuronale Erregbarkeit reduziert und vor Exzitotoxizität geschützt wird . Darüber hinaus moduliert es die Expression spezifischer Micro-RNAs im Hippocampus, die an den in Tiermodellen beobachteten antidepressiven Wirkungen beteiligt sind .
Vorbereitungsmethoden
The synthesis of 6-chlorokynurenic acid typically involves several steps:
Cyclization Reaction: Starting with low-cost DL-malic acid, a cyclization reaction is carried out to form a precursor compound.
Ammonification Reaction: The precursor undergoes an ammonification reaction to yield 6-hydroxynicotinic acid.
Chlorination Reaction: Finally, 6-hydroxynicotinic acid is subjected to a chlorination reaction to produce 6-chlorokynurenic acid
These reactions are performed under relatively simple conditions, with minimal environmental impact and high yield, making the process economically viable for industrial production .
Analyse Chemischer Reaktionen
6-Chlorokynurenic acid undergoes several types of chemical reactions:
Oxidation: It can be oxidized to form various quinolinic acid derivatives.
Reduction: Reduction reactions can modify the quinoline ring structure.
Substitution: The chlorine atom at position 6 can be substituted with other functional groups under appropriate conditions.
Common reagents used in these reactions include oxidizing agents like hypochlorous acid and reducing agents like sodium borohydride . The major products formed from these reactions are typically other quinolinic acid derivatives with modified functional groups .
Wissenschaftliche Forschungsanwendungen
6-Chlorokynurenic acid has a wide range of scientific research applications:
Neuroprotection: It acts as a neuroprotective agent by antagonizing the NMDA receptor, preventing neurodegeneration caused by excitotoxicity.
Antidepressant Effects: It has been shown to produce rapid antidepressant-like effects by regulating hippocampal microRNA expressions involved in the TrkB-ERK/Akt signaling pathways.
Glutamate Reuptake Inhibition: It inhibits the reuptake of glutamate into synaptic vesicles, thereby modulating synaptic transmission.
Wirkmechanismus
6-Chlorokynurenic acid exerts its effects primarily through antagonism of the NMDA receptor at the glycine site. This inhibition prevents the excitatory neurotransmitter glutamate from binding to the receptor, thereby reducing neuronal excitability and protecting against excitotoxicity . Additionally, it modulates the expression of specific microRNAs in the hippocampus, which are involved in the antidepressant effects observed in animal models .
Vergleich Mit ähnlichen Verbindungen
6-Chlorokynurensäure wird häufig mit anderen Kynurensäurederivaten verglichen:
7-Chlorokynurensäure: Ähnlich in der Struktur, jedoch mit dem Chloratom an Position 7.
5,7-Dichlorokynurensäure: Enthält zwei Chloratome an den Positionen 5 und 7.
4-Chlorokynurenin: Ein Prodrug, das im Gehirn in 6-Chlorokynurensäure umgewandelt wird und so die Übertragung über die Blut-Hirn-Schranke erleichtert.
Diese Verbindungen unterstreichen die einzigartigen Eigenschaften von 6-Chlorokynurensäure, insbesondere das Gleichgewicht zwischen Potenz und Hirndurchdringung .
Biologische Aktivität
6-Chloro-4-oxo-1,4-dihydroquinoline-2-carboxylic acid is a notable compound in medicinal chemistry, primarily recognized for its diverse biological activities, including antimicrobial and potential anticancer properties. This article explores the compound's biological activity, mechanisms of action, and relevant research findings.
Chemical Structure and Properties
The compound has the molecular formula and a molecular weight of approximately 223.61 g/mol. Its structure features a fused quinoline system with a chloro substituent at the 6-position and a carboxylic acid group at the 2-position, which are critical for its biological reactivity and pharmacological effects.
Inhibition of Bacterial Enzymes :
this compound primarily exerts its antibacterial effects by inhibiting bacterial DNA gyrase and topoisomerase IV. These enzymes are essential for bacterial DNA replication and repair. By disrupting these processes, the compound leads to cell death in susceptible bacteria.
Cellular Effects :
The compound induces significant cellular responses in bacteria, including the SOS response, which activates genes involved in DNA repair and cell cycle regulation. This effect highlights its role in modulating bacterial stress responses.
Antimicrobial Activity
Research has demonstrated that this compound exhibits potent antimicrobial activity against various bacterial strains. Its efficacy is particularly noted against Gram-negative bacteria due to its ability to penetrate bacterial membranes effectively.
Bacterial Strain | Minimum Inhibitory Concentration (MIC) |
---|---|
Escherichia coli | 0.5 µg/mL |
Staphylococcus aureus | 1.0 µg/mL |
Pseudomonas aeruginosa | 2.0 µg/mL |
Antiviral Activity
In addition to its antibacterial properties, this compound has shown potential antiviral activity. A related compound, 6-chloro-1,4-dihydro-4-oxo-1-(beta-D-ribofuranosyl) quinoline-3-carboxylic acid, was found to inhibit HIV-1 replication in human primary cells with an EC50 of approximately 1.5 µM . This suggests that derivatives of the quinoline family may have broader antiviral applications.
Case Studies and Research Findings
A significant study highlighted the synthesis of various derivatives of this compound, exploring their biological activities through structure-activity relationship (SAR) analysis. Modifications at different positions on the quinoline ring led to enhanced antimicrobial potency and selectivity against specific pathogens .
Example Case Study: Synthesis and Evaluation
A recent study synthesized several derivatives of this compound using various amines and evaluated their biological activities against a panel of bacterial strains. The results indicated that certain substitutions significantly improved antimicrobial effectiveness:
Derivative | Activity | MIC (µg/mL) |
---|---|---|
3-Amino derivative | Enhanced activity | 0.25 |
5-Methyl derivative | Moderate activity | 0.75 |
Non-substituted parent compound | Baseline activity | 1.0 |
Eigenschaften
IUPAC Name |
6-chloro-4-oxo-1H-quinoline-2-carboxylic acid | |
---|---|---|
Source | PubChem | |
URL | https://pubchem.ncbi.nlm.nih.gov | |
Description | Data deposited in or computed by PubChem | |
InChI |
InChI=1S/C10H6ClNO3/c11-5-1-2-7-6(3-5)9(13)4-8(12-7)10(14)15/h1-4H,(H,12,13)(H,14,15) | |
Source | PubChem | |
URL | https://pubchem.ncbi.nlm.nih.gov | |
Description | Data deposited in or computed by PubChem | |
InChI Key |
FBHYBXRWVNVMRY-UHFFFAOYSA-N | |
Source | PubChem | |
URL | https://pubchem.ncbi.nlm.nih.gov | |
Description | Data deposited in or computed by PubChem | |
Canonical SMILES |
C1=CC2=C(C=C1Cl)C(=O)C=C(N2)C(=O)O | |
Source | PubChem | |
URL | https://pubchem.ncbi.nlm.nih.gov | |
Description | Data deposited in or computed by PubChem | |
Molecular Formula |
C10H6ClNO3 | |
Source | PubChem | |
URL | https://pubchem.ncbi.nlm.nih.gov | |
Description | Data deposited in or computed by PubChem | |
DSSTOX Substance ID |
DTXSID90906582 | |
Record name | 6-Chloro-4-oxo-1,4-dihydroquinoline-2-carboxylic acid | |
Source | EPA DSSTox | |
URL | https://comptox.epa.gov/dashboard/DTXSID90906582 | |
Description | DSSTox provides a high quality public chemistry resource for supporting improved predictive toxicology. | |
Molecular Weight |
223.61 g/mol | |
Source | PubChem | |
URL | https://pubchem.ncbi.nlm.nih.gov | |
Description | Data deposited in or computed by PubChem | |
CAS No. |
10174-72-8 | |
Record name | 10174-72-8 | |
Source | DTP/NCI | |
URL | https://dtp.cancer.gov/dtpstandard/servlet/dwindex?searchtype=NSC&outputformat=html&searchlist=403023 | |
Description | The NCI Development Therapeutics Program (DTP) provides services and resources to the academic and private-sector research communities worldwide to facilitate the discovery and development of new cancer therapeutic agents. | |
Explanation | Unless otherwise indicated, all text within NCI products is free of copyright and may be reused without our permission. Credit the National Cancer Institute as the source. | |
Record name | 6-Chloro-4-oxo-1,4-dihydroquinoline-2-carboxylic acid | |
Source | EPA DSSTox | |
URL | https://comptox.epa.gov/dashboard/DTXSID90906582 | |
Description | DSSTox provides a high quality public chemistry resource for supporting improved predictive toxicology. | |
Retrosynthesis Analysis
AI-Powered Synthesis Planning: Our tool employs the Template_relevance Pistachio, Template_relevance Bkms_metabolic, Template_relevance Pistachio_ringbreaker, Template_relevance Reaxys, Template_relevance Reaxys_biocatalysis model, leveraging a vast database of chemical reactions to predict feasible synthetic routes.
One-Step Synthesis Focus: Specifically designed for one-step synthesis, it provides concise and direct routes for your target compounds, streamlining the synthesis process.
Accurate Predictions: Utilizing the extensive PISTACHIO, BKMS_METABOLIC, PISTACHIO_RINGBREAKER, REAXYS, REAXYS_BIOCATALYSIS database, our tool offers high-accuracy predictions, reflecting the latest in chemical research and data.
Strategy Settings
Precursor scoring | Relevance Heuristic |
---|---|
Min. plausibility | 0.01 |
Model | Template_relevance |
Template Set | Pistachio/Bkms_metabolic/Pistachio_ringbreaker/Reaxys/Reaxys_biocatalysis |
Top-N result to add to graph | 6 |
Feasible Synthetic Routes
Haftungsausschluss und Informationen zu In-Vitro-Forschungsprodukten
Bitte beachten Sie, dass alle Artikel und Produktinformationen, die auf BenchChem präsentiert werden, ausschließlich zu Informationszwecken bestimmt sind. Die auf BenchChem zum Kauf angebotenen Produkte sind speziell für In-vitro-Studien konzipiert, die außerhalb lebender Organismen durchgeführt werden. In-vitro-Studien, abgeleitet von dem lateinischen Begriff "in Glas", beinhalten Experimente, die in kontrollierten Laborumgebungen unter Verwendung von Zellen oder Geweben durchgeführt werden. Es ist wichtig zu beachten, dass diese Produkte nicht als Arzneimittel oder Medikamente eingestuft sind und keine Zulassung der FDA für die Vorbeugung, Behandlung oder Heilung von medizinischen Zuständen, Beschwerden oder Krankheiten erhalten haben. Wir müssen betonen, dass jede Form der körperlichen Einführung dieser Produkte in Menschen oder Tiere gesetzlich strikt untersagt ist. Es ist unerlässlich, sich an diese Richtlinien zu halten, um die Einhaltung rechtlicher und ethischer Standards in Forschung und Experiment zu gewährleisten.