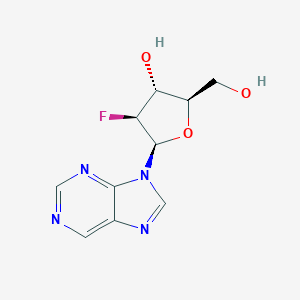
(2R,3R,4S,5R)-4-Fluoro-2-(hydroxymethyl)-5-(9H-purin-9-yl)tetrahydrofuran-3-ol
- Klicken Sie auf QUICK INQUIRY, um ein Angebot von unserem Expertenteam zu erhalten.
- Mit qualitativ hochwertigen Produkten zu einem WETTBEWERBSFÄHIGEN Preis können Sie sich mehr auf Ihre Forschung konzentrieren.
Übersicht
Beschreibung
(2R,3R,4S,5R)-4-Fluoro-2-(hydroxymethyl)-5-(9H-purin-9-yl)tetrahydrofuran-3-ol is a synthetic nucleoside analog. This compound is structurally related to naturally occurring nucleosides but has been modified to include a fluorine atom and other functional groups. These modifications can significantly alter its biological activity, making it a valuable molecule in medicinal chemistry and drug development.
Vorbereitungsmethoden
Synthetic Routes and Reaction Conditions
The synthesis of (2R,3R,4S,5R)-4-Fluoro-2-(hydroxymethyl)-5-(9H-purin-9-yl)tetrahydrofuran-3-ol typically involves several key steps:
Starting Materials: The synthesis begins with a suitable sugar derivative, such as a protected ribose or deoxyribose.
Fluorination: Introduction of the fluorine atom is achieved through nucleophilic substitution reactions using reagents like diethylaminosulfur trifluoride (DAST) or similar fluorinating agents.
Glycosylation: The fluorinated sugar is then coupled with a purine base, such as adenine, using glycosylation reactions. This step often requires the use of Lewis acids like trimethylsilyl trifluoromethanesulfonate (TMSOTf) to activate the sugar.
Deprotection: Finally, any protecting groups on the sugar or base are removed under acidic or basic conditions to yield the desired nucleoside analog.
Industrial Production Methods
Industrial production of this compound would follow similar synthetic routes but on a larger scale. Key considerations include:
Optimization of Reaction Conditions: Ensuring high yield and purity through controlled reaction conditions.
Scalability: Using batch or continuous flow processes to produce large quantities.
Purification: Employing techniques such as crystallization, chromatography, and recrystallization to achieve the desired purity.
Analyse Chemischer Reaktionen
Types of Reactions
Oxidation: The hydroxymethyl group can undergo oxidation to form aldehydes or carboxylic acids.
Reduction: The compound can be reduced to modify the purine base or the sugar moiety.
Substitution: The fluorine atom can be substituted with other nucleophiles under appropriate conditions.
Common Reagents and Conditions
Oxidation: Reagents like potassium permanganate (KMnO₄) or chromium trioxide (CrO₃) in acidic conditions.
Reduction: Hydrogenation using palladium on carbon (Pd/C) or sodium borohydride (NaBH₄).
Substitution: Nucleophiles such as thiols, amines, or halides in the presence of catalysts or under thermal conditions.
Major Products
Oxidation: Formation of 2-(hydroxymethyl)-5-(9H-purin-9-yl)tetrahydrofuran-3-one.
Reduction: Formation of 2-(hydroxymethyl)-5-(9H-purin-9-yl)tetrahydrofuran-3-amine.
Substitution: Formation of 4-substituted derivatives depending on the nucleophile used.
Wissenschaftliche Forschungsanwendungen
Chemistry
Synthesis of Modified Nucleosides: Used as building blocks for the synthesis of more complex nucleic acid analogs.
Chemical Probes: Employed in studying enzyme mechanisms and interactions with nucleic acids.
Biology
Antiviral Agents: Investigated for its potential to inhibit viral replication by incorporating into viral DNA or RNA.
Enzyme Inhibitors: Studied as inhibitors of enzymes involved in nucleic acid metabolism.
Medicine
Anticancer Agents: Explored for its ability to interfere with DNA synthesis in rapidly dividing cancer cells.
Diagnostic Tools: Used in imaging and diagnostic assays to detect specific nucleic acid sequences.
Industry
Pharmaceuticals: Incorporated into drug formulations for targeted therapies.
Biotechnology: Utilized in the development of nucleic acid-based technologies and products.
Wirkmechanismus
The mechanism of action of (2R,3R,4S,5R)-4-Fluoro-2-(hydroxymethyl)-5-(9H-purin-9-yl)tetrahydrofuran-3-ol involves its incorporation into nucleic acids. Once incorporated, it can:
Inhibit DNA/RNA Polymerases: By acting as a chain terminator during nucleic acid synthesis.
Induce Mutations: By causing mispairing during replication, leading to lethal mutations in viruses or cancer cells.
Enzyme Inhibition: By binding to and inhibiting enzymes involved in nucleic acid metabolism.
Vergleich Mit ähnlichen Verbindungen
Similar Compounds
2’-Fluoro-2’-deoxyadenosine: Another fluorinated nucleoside analog with similar antiviral and anticancer properties.
2’-C-Methyladenosine: A nucleoside analog used in antiviral therapies.
3’-Deoxyadenosine: Known for its role in inhibiting DNA synthesis.
Uniqueness
(2R,3R,4S,5R)-4-Fluoro-2-(hydroxymethyl)-5-(9H-purin-9-yl)tetrahydrofuran-3-ol is unique due to its specific stereochemistry and the presence of both a fluorine atom and a hydroxymethyl group. These features confer distinct biological activities and make it a versatile tool in medicinal chemistry.
This detailed overview should provide a comprehensive understanding of this compound, its synthesis, reactions, applications, and unique properties
Eigenschaften
IUPAC Name |
(2R,3R,4S,5R)-4-fluoro-2-(hydroxymethyl)-5-purin-9-yloxolan-3-ol |
Source
|
---|---|---|
Source | PubChem | |
URL | https://pubchem.ncbi.nlm.nih.gov | |
Description | Data deposited in or computed by PubChem | |
InChI |
InChI=1S/C10H11FN4O3/c11-7-8(17)6(2-16)18-10(7)15-4-14-5-1-12-3-13-9(5)15/h1,3-4,6-8,10,16-17H,2H2/t6-,7+,8-,10-/m1/s1 |
Source
|
Source | PubChem | |
URL | https://pubchem.ncbi.nlm.nih.gov | |
Description | Data deposited in or computed by PubChem | |
InChI Key |
HLBLWLNFMONEHG-IBCQBUCCSA-N |
Source
|
Source | PubChem | |
URL | https://pubchem.ncbi.nlm.nih.gov | |
Description | Data deposited in or computed by PubChem | |
Canonical SMILES |
C1=C2C(=NC=N1)N(C=N2)C3C(C(C(O3)CO)O)F |
Source
|
Source | PubChem | |
URL | https://pubchem.ncbi.nlm.nih.gov | |
Description | Data deposited in or computed by PubChem | |
Isomeric SMILES |
C1=C2C(=NC=N1)N(C=N2)[C@H]3[C@H]([C@@H]([C@H](O3)CO)O)F |
Source
|
Source | PubChem | |
URL | https://pubchem.ncbi.nlm.nih.gov | |
Description | Data deposited in or computed by PubChem | |
Molecular Formula |
C10H11FN4O3 |
Source
|
Source | PubChem | |
URL | https://pubchem.ncbi.nlm.nih.gov | |
Description | Data deposited in or computed by PubChem | |
Molecular Weight |
254.22 g/mol |
Source
|
Source | PubChem | |
URL | https://pubchem.ncbi.nlm.nih.gov | |
Description | Data deposited in or computed by PubChem | |
Haftungsausschluss und Informationen zu In-Vitro-Forschungsprodukten
Bitte beachten Sie, dass alle Artikel und Produktinformationen, die auf BenchChem präsentiert werden, ausschließlich zu Informationszwecken bestimmt sind. Die auf BenchChem zum Kauf angebotenen Produkte sind speziell für In-vitro-Studien konzipiert, die außerhalb lebender Organismen durchgeführt werden. In-vitro-Studien, abgeleitet von dem lateinischen Begriff "in Glas", beinhalten Experimente, die in kontrollierten Laborumgebungen unter Verwendung von Zellen oder Geweben durchgeführt werden. Es ist wichtig zu beachten, dass diese Produkte nicht als Arzneimittel oder Medikamente eingestuft sind und keine Zulassung der FDA für die Vorbeugung, Behandlung oder Heilung von medizinischen Zuständen, Beschwerden oder Krankheiten erhalten haben. Wir müssen betonen, dass jede Form der körperlichen Einführung dieser Produkte in Menschen oder Tiere gesetzlich strikt untersagt ist. Es ist unerlässlich, sich an diese Richtlinien zu halten, um die Einhaltung rechtlicher und ethischer Standards in Forschung und Experiment zu gewährleisten.