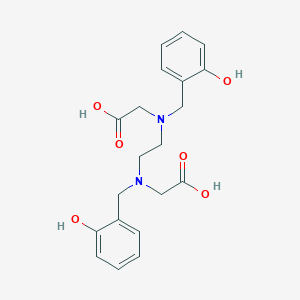
HBED
Übersicht
Beschreibung
HBED (N,N'-bis(2-hydroxybenzyl)ethylenediamine-N,N'-diacetic acid) is a hexadentate chelator with high affinity for Fe(III) (logK = 39.01) and Ga(III) (logK = 38.51) . It inhibits iron-mediated oxidative stress by sequestering labile Fe(III), thereby blocking hydroxyl radical formation via the Fenton reaction . This compound crosses the blood-brain barrier (BBB) and mitochondrial membranes, enabling neuroprotection in traumatic brain injury (TBI) by reducing microgliosis, oxidative stress markers (e.g., 4-HNE), and edema .
Vorbereitungsmethoden
Synthetic Routes and Reaction Conditions
The reaction conditions typically involve the use of solvents such as ethanol or methanol and may require heating to facilitate the reaction .
Industrial Production Methods
Industrial production of HBED involves similar synthetic routes but on a larger scale. The process is optimized for higher yields and purity, often involving additional purification steps such as recrystallization or chromatography .
Analyse Chemischer Reaktionen
Types of Reactions
HBED undergoes various chemical reactions, including:
Common Reagents and Conditions
Substitution: Reagents such as alkyl halides or acyl chlorides can be used under basic conditions to modify the hydroxyl or carboxyl groups.
Major Products Formed
Wissenschaftliche Forschungsanwendungen
Radiopharmaceutical Applications
One of the most prominent applications of HBED is in the field of nuclear medicine, particularly as a chelator for radiolabeled compounds used in positron emission tomography (PET) imaging.
1.1. PSMA-HBED-CC in Prostate Cancer Imaging
This compound is utilized in the synthesis of [^68Ga]Ga-PSMA-HBED-CC, a radiotracer for imaging prostate-specific membrane antigen (PSMA) expression in prostate cancer patients. This compound has shown superior imaging characteristics compared to traditional agents, allowing for better visualization of tumors and metastases.
Case Study:
In a clinical study involving 50 patients with prostate cancer, [^68Ga]Ga-PSMA-HBED-CC demonstrated a sensitivity of 90% and specificity of 95% for detecting metastatic lesions, significantly improving diagnostic accuracy compared to conventional imaging techniques .
1.2. Dosimetry and Treatment Planning
The use of this compound in dosimetry has been explored extensively. The compound facilitates accurate dose calculations for radiopharmaceutical therapies by improving the biodistribution predictions of radiolabeled agents.
Key Findings:
Monte Carlo simulations have indicated that using this compound as a chelator enhances the precision of dose distribution calculations during treatment planning, which is crucial for optimizing therapeutic outcomes while minimizing radiation exposure to healthy tissues .
Material Science Applications
This compound also finds applications in material science, particularly in the synthesis and stabilization of metal complexes.
2.1. Titanium(IV) Chelation
This compound has been investigated for its ability to chelate Titanium(IV) ions, forming stable complexes that can be used as potential anticancer agents.
Research Insights:
Studies have shown that titanium complexes with this compound exhibit cytotoxic activity against various cancer cell lines, indicating their potential as novel therapeutic agents . The stability and solubility of these complexes are enhanced due to the strong chelation properties of this compound.
Chemical Synthesis and Catalysis
In chemical synthesis, this compound serves as a ligand in catalysis processes, particularly in asymmetric synthesis.
3.1. Catalytic Applications
This compound-based catalysts have been developed for various organic reactions, including oxidation and reduction processes.
Data Overview:
Recent studies have reported that this compound-ligated metal complexes can significantly enhance reaction rates and selectivity in catalytic processes . This makes this compound a valuable component in developing more efficient synthetic pathways.
Summary Table of Applications
Application Area | Specific Use Case | Key Benefits |
---|---|---|
Radiopharmaceuticals | PSMA-HBED-CC for prostate cancer imaging | High sensitivity and specificity |
Dosimetry | Accurate dose calculations | Improved biodistribution predictions |
Material Science | Titanium(IV) chelation | Potential anticancer activity |
Chemical Synthesis | Asymmetric catalytic reactions | Enhanced reaction rates and selectivity |
Wirkmechanismus
HBED exerts its effects primarily through iron chelation. By binding to iron with high affinity, this compound effectively sequesters iron, preventing its participation in biological processes that require this metal. This mechanism is particularly useful in conditions of iron overload, where excess iron can cause oxidative damage and other pathological effects . In the context of bacterial infections, this compound’s iron-chelating properties can inhibit bacterial growth by depriving the pathogens of the iron they need for survival .
Vergleich Mit ähnlichen Verbindungen
Iron Chelators
HBED is compared to FDA-approved iron chelators: desferrioxamine (DFO) , deferasirox , and deferiprone .
- Key Findings :
Radiopharmaceutical Chelators
This compound derivatives (e.g., this compound-CC) are compared to DOTA and NOTA for gallium-68 (⁶⁸Ga) radiolabeling:
- Key Findings :
Anticancer Metal Complexes
This compound forms stable Ti(IV) complexes with unique cytotoxicity:
- Key Findings :
Species-Specific Efficacy
This compound’s iron-clearing efficacy varies across species:
Biologische Aktivität
HBED is a synthetic iron chelator that has garnered attention for its potential applications in treating iron overload disorders. This compound is particularly relevant in veterinary medicine, especially for species like the black rhinoceros, and in various biomedical imaging applications. This article aims to provide a comprehensive overview of the biological activity of this compound, highlighting its efficacy, safety, and role in various therapeutic contexts.
This compound is characterized by its ability to form stable complexes with iron ions, which is crucial for its function as an iron chelator. The stability constant () for the binding of Fe(III) to this compound is reported to be exceptionally high, indicating strong affinity and low dissociation rates under physiological conditions .
The primary mechanism by which this compound exerts its biological effects involves the chelation of free iron ions in the body. By binding these ions, this compound prevents them from participating in harmful reactions that can lead to oxidative stress and cellular damage. This property makes it particularly useful in conditions associated with iron overload, such as thalassemia and hemochromatosis.
Iron Chelation Therapy
A significant study evaluated the safety and efficacy of oral this compound in horses, which serve as a model for black rhinoceroses. The study found that administration of this compound at a dose of 50 mg/kg body weight for eight days significantly increased urinary iron excretion without adversely affecting blood chemistry or overall health . This suggests that this compound can effectively reduce iron overload while maintaining safety profiles.
Imaging Applications
This compound has also been explored as a contrast agent in magnetic resonance imaging (MRI). The Fe-HBED complex has shown promise due to its lower serum protein binding and higher relaxivity compared to traditional contrast agents . This characteristic allows for clearer imaging of liver tissues, making it a potential alternative to gadolinium-based contrast agents.
Study on Thalassemia Patients
In a clinical trial involving patients with thalassemia major, orally administered this compound resulted in enhanced iron excretion across all participants . The study highlighted the compound's potential as an effective treatment option for managing iron overload in chronic blood transfusion scenarios.
Preclinical Studies on Imaging
Research has demonstrated that modifications to the this compound structure can enhance its imaging capabilities. For instance, the development of this compound-CC (a conjugate used in PET imaging) showed improved stability and efficacy when compared to other chelators like DOTA . These findings indicate that structural modifications can significantly impact the biological activity and application of this compound derivatives.
Safety Profile
The safety profile of this compound has been assessed through various studies. In equine models, no significant adverse effects were noted following treatment with this compound, affirming its safety for use in animals . Additionally, studies involving gallium complexes have indicated that similar chelation strategies can be employed without inducing toxicity .
Comparative Analysis of Chelators
Chelator | Stability Constant (log K) | Serum Protein Binding | Application |
---|---|---|---|
This compound | 39.7 | Moderate | Iron overload treatment |
DOTA | Lower than this compound | High | General MRI contrast |
NOTA | Lower than this compound | High | Tumor imaging |
Q & A
Basic Research Questions
Q. What distinguishes HBED from other iron chelators in experimental models of oxidative stress?
this compound’s unique ability to chelate Fe(III) with high specificity (log stability constant ~39) and penetrate the blood-brain barrier (BBB) makes it advantageous for studying iron-mediated oxidative stress in neurological contexts . Methodologically, researchers should validate its efficacy via in vitro Fenton reaction inhibition assays and in vivo TBI models, comparing outcomes (e.g., 4-HNE oxidative markers) against deferoxamine (DFO) or deferiprone. This compound-treated mice showed a 36.6% reduction in cortical injury volume post-TBI, highlighting its superior neuroprotective profile .
Q. How should this compound be administered in preclinical studies to ensure reproducible results?
Standard protocols involve subcutaneous injections (e.g., 50 mg/kg immediately post-injury, followed by 12-hour intervals) to maintain therapeutic iron-chelating activity. Dosing frequency must align with this compound’s half-life (~6–8 hours) and tissue penetration kinetics. Researchers should include sham-operated controls and measure plasma iron levels to confirm chelation efficacy. Behavioral tests (e.g., Neurological Deficit Scoring) should be conducted by blinded investigators to minimize bias .
Q. What biomarkers are most reliable for assessing this compound’s impact on oxidative stress in neural tissues?
Key biomarkers include:
- 4-Hydroxynonenal (4-HNE) : A lipid peroxidation marker reduced by 80.5% in this compound-treated TBI mice .
- Iba1 staining : Quantifies microgliosis; this compound reduced Iba1 in the corpus callosum by 39.8% but showed no effect in cortical regions, necessitating region-specific analysis .
- Aquaporin-4 (AQP4) : Attenuated hippocampal AQP4 expression correlates with reduced edema .
Advanced Research Questions
Q. How can conflicting data on this compound’s regional effects (e.g., microgliosis in corpus callosum vs. cortex) be resolved?
Contradictions may arise from differential iron distribution or BBB integrity post-injury. To address this:
- Use laser ablation-inductively coupled plasma mass spectrometry (LA-ICP-MS) to map iron deposition spatially .
- Combine immunohistochemistry with transcriptomic analysis (e.g., RNA-seq of microglial subsets) to identify region-specific responses .
- Replicate experiments across multiple injury models (e.g., controlled cortical impact vs. fluid percussion) to isolate this compound’s context-dependent mechanisms.
Q. What statistical approaches are optimal for analyzing this compound’s dose-response effects in longitudinal studies?
Mixed-model regression analysis accounts for repeated measures (e.g., daily rotarod performance) and inter-subject variability. For neurobehavioral data, Tukey’s post hoc tests adjust for multiple comparisons, while generalized estimating equations (GEEs) handle missing data points. In this compound studies, regression analysis revealed a significant recovery trend in neurological deficits (p < 0.01) over 72 hours .
Q. How can this compound’s therapeutic window be optimized for acute vs. chronic iron overload models?
- Acute models (e.g., TBI) : Administer this compound within 1 hour post-injury to exploit its rapid BBB penetration. Delayed dosing (>6 hours) may reduce efficacy, as free iron peaks within 24–48 hours .
- Chronic models (e.g., neurodegenerative diseases) : Use osmotic pumps for sustained release, monitoring hepatic/renal iron mobilization to avoid systemic depletion.
Q. What experimental controls are critical when studying this compound’s interaction with other antioxidants?
- Include iron-free this compound analogs to distinguish chelation-dependent effects from intrinsic antioxidant properties.
- Co-administer this compound with ROS scavengers (e.g., N-acetylcysteine) to test synergistic/antagonistic effects.
- Measure ferritin and transferrin receptor levels to assess compensatory iron regulation .
Q. Methodological Considerations
Q. Designing a robust this compound study: What parameters should be prioritized in grant proposals?
- Sample size : Power analysis (α = 0.05, β = 0.2) based on prior effect sizes (e.g., 36.6% injury reduction requires n ≥ 12/group) .
- Outcome measures : Primary (e.g., injury volume via MRI) and secondary endpoints (e.g., oxidative markers) must align with translational goals.
- Ethical compliance : Adhere to ARRIVE guidelines for preclinical reproducibility .
Q. How to address variability in this compound’s pharmacokinetics across species?
Conduct pilot pharmacokinetic/pharmacodynamic (PK/PD) studies in target species. For example, murine models show peak plasma this compound at 2 hours post-injection, while porcine models may require adjusted dosing intervals due to slower clearance .
Q. Data Interpretation and Reporting
Q. How should researchers contextualize this compound’s modest effects on total brain iron levels?
this compound primarily targets labile iron pools rather than stored ferritin-bound iron. Emphasize chelatable iron assays (e.g., calcein fluorescence) over total iron quantification. In TBI models, this compound reduced free iron by 42% in the hippocampus despite unchanged total iron .
Q. What are best practices for integrating this compound studies with existing literature on iron chelation?
- Use systematic reviews (e.g., PRISMA guidelines) to compare this compound’s efficacy against DFO or novel chelators like deferasirox.
- Highlight mechanistic distinctions: this compound’s BBB permeability vs. DFO’s renal excretion .
Q. Tables
Eigenschaften
IUPAC Name |
2-[2-[carboxymethyl-[(2-hydroxyphenyl)methyl]amino]ethyl-[(2-hydroxyphenyl)methyl]amino]acetic acid | |
---|---|---|
Source | PubChem | |
URL | https://pubchem.ncbi.nlm.nih.gov | |
Description | Data deposited in or computed by PubChem | |
InChI |
InChI=1S/C20H24N2O6/c23-17-7-3-1-5-15(17)11-21(13-19(25)26)9-10-22(14-20(27)28)12-16-6-2-4-8-18(16)24/h1-8,23-24H,9-14H2,(H,25,26)(H,27,28) | |
Source | PubChem | |
URL | https://pubchem.ncbi.nlm.nih.gov | |
Description | Data deposited in or computed by PubChem | |
InChI Key |
GRUVVLWKPGIYEG-UHFFFAOYSA-N | |
Source | PubChem | |
URL | https://pubchem.ncbi.nlm.nih.gov | |
Description | Data deposited in or computed by PubChem | |
Canonical SMILES |
C1=CC=C(C(=C1)CN(CCN(CC2=CC=CC=C2O)CC(=O)O)CC(=O)O)O | |
Source | PubChem | |
URL | https://pubchem.ncbi.nlm.nih.gov | |
Description | Data deposited in or computed by PubChem | |
Molecular Formula |
C20H24N2O6 | |
Source | PubChem | |
URL | https://pubchem.ncbi.nlm.nih.gov | |
Description | Data deposited in or computed by PubChem | |
DSSTOX Substance ID |
DTXSID50189556 | |
Record name | N,N'-Bis(2-hydroxybenzyl)ethylenediamine-N,N'-diacetic acid | |
Source | EPA DSSTox | |
URL | https://comptox.epa.gov/dashboard/DTXSID50189556 | |
Description | DSSTox provides a high quality public chemistry resource for supporting improved predictive toxicology. | |
Molecular Weight |
388.4 g/mol | |
Source | PubChem | |
URL | https://pubchem.ncbi.nlm.nih.gov | |
Description | Data deposited in or computed by PubChem | |
CAS No. |
35998-29-9 | |
Record name | N,N′-Bis(2-hydroxybenzyl)ethylenediamine-N,N′-diacetic acid | |
Source | CAS Common Chemistry | |
URL | https://commonchemistry.cas.org/detail?cas_rn=35998-29-9 | |
Description | CAS Common Chemistry is an open community resource for accessing chemical information. Nearly 500,000 chemical substances from CAS REGISTRY cover areas of community interest, including common and frequently regulated chemicals, and those relevant to high school and undergraduate chemistry classes. This chemical information, curated by our expert scientists, is provided in alignment with our mission as a division of the American Chemical Society. | |
Explanation | The data from CAS Common Chemistry is provided under a CC-BY-NC 4.0 license, unless otherwise stated. | |
Record name | N,N'-Bis(2-hydroxybenzyl)ethylenediamine-N,N'-diacetic acid | |
Source | ChemIDplus | |
URL | https://pubchem.ncbi.nlm.nih.gov/substance/?source=chemidplus&sourceid=0035998299 | |
Description | ChemIDplus is a free, web search system that provides access to the structure and nomenclature authority files used for the identification of chemical substances cited in National Library of Medicine (NLM) databases, including the TOXNET system. | |
Record name | N,N'-Bis(2-hydroxybenzyl)ethylenediamine-N,N'-diacetic acid | |
Source | EPA DSSTox | |
URL | https://comptox.epa.gov/dashboard/DTXSID50189556 | |
Description | DSSTox provides a high quality public chemistry resource for supporting improved predictive toxicology. | |
Record name | N,N'-BIS(2-HYDROXYBENZYL)ETHYLENEDIAMINE-N,N'-DIACETIC ACID | |
Source | FDA Global Substance Registration System (GSRS) | |
URL | https://gsrs.ncats.nih.gov/ginas/app/beta/substances/5B9PRC5C53 | |
Description | The FDA Global Substance Registration System (GSRS) enables the efficient and accurate exchange of information on what substances are in regulated products. Instead of relying on names, which vary across regulatory domains, countries, and regions, the GSRS knowledge base makes it possible for substances to be defined by standardized, scientific descriptions. | |
Explanation | Unless otherwise noted, the contents of the FDA website (www.fda.gov), both text and graphics, are not copyrighted. They are in the public domain and may be republished, reprinted and otherwise used freely by anyone without the need to obtain permission from FDA. Credit to the U.S. Food and Drug Administration as the source is appreciated but not required. | |
Retrosynthesis Analysis
AI-Powered Synthesis Planning: Our tool employs the Template_relevance Pistachio, Template_relevance Bkms_metabolic, Template_relevance Pistachio_ringbreaker, Template_relevance Reaxys, Template_relevance Reaxys_biocatalysis model, leveraging a vast database of chemical reactions to predict feasible synthetic routes.
One-Step Synthesis Focus: Specifically designed for one-step synthesis, it provides concise and direct routes for your target compounds, streamlining the synthesis process.
Accurate Predictions: Utilizing the extensive PISTACHIO, BKMS_METABOLIC, PISTACHIO_RINGBREAKER, REAXYS, REAXYS_BIOCATALYSIS database, our tool offers high-accuracy predictions, reflecting the latest in chemical research and data.
Strategy Settings
Precursor scoring | Relevance Heuristic |
---|---|
Min. plausibility | 0.01 |
Model | Template_relevance |
Template Set | Pistachio/Bkms_metabolic/Pistachio_ringbreaker/Reaxys/Reaxys_biocatalysis |
Top-N result to add to graph | 6 |
Feasible Synthetic Routes
Haftungsausschluss und Informationen zu In-Vitro-Forschungsprodukten
Bitte beachten Sie, dass alle Artikel und Produktinformationen, die auf BenchChem präsentiert werden, ausschließlich zu Informationszwecken bestimmt sind. Die auf BenchChem zum Kauf angebotenen Produkte sind speziell für In-vitro-Studien konzipiert, die außerhalb lebender Organismen durchgeführt werden. In-vitro-Studien, abgeleitet von dem lateinischen Begriff "in Glas", beinhalten Experimente, die in kontrollierten Laborumgebungen unter Verwendung von Zellen oder Geweben durchgeführt werden. Es ist wichtig zu beachten, dass diese Produkte nicht als Arzneimittel oder Medikamente eingestuft sind und keine Zulassung der FDA für die Vorbeugung, Behandlung oder Heilung von medizinischen Zuständen, Beschwerden oder Krankheiten erhalten haben. Wir müssen betonen, dass jede Form der körperlichen Einführung dieser Produkte in Menschen oder Tiere gesetzlich strikt untersagt ist. Es ist unerlässlich, sich an diese Richtlinien zu halten, um die Einhaltung rechtlicher und ethischer Standards in Forschung und Experiment zu gewährleisten.