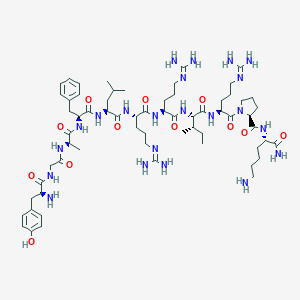
H-Tyr-Gly-D-Ala-Phe-Leu-Arg-Arg-Ile-Arg-Pro-Lys-NH2
Übersicht
Beschreibung
The peptide features a 12-residue sequence with key modifications:
- D-Ala at position 3: Enhances metabolic stability by resisting enzymatic degradation, a common strategy in peptide design .
- Arg-rich motif (Arg⁶-Arg⁷-Ile⁸-Arg⁹): Likely critical for receptor binding, as arginine residues contribute to cationicity and electrostatic interactions with receptors .
- C-terminal Pro-Lys-NH₂: A conserved motif in dynorphin analogs, stabilizing secondary structure and receptor affinity .
While its exact pharmacological profile remains understudied, its structural homology to dynorphin fragments (e.g., Dyn A-(1-11)NH₂) suggests κ-opioid receptor (KOR) activity .
Vorbereitungsmethoden
Synthetic Routes and Reaction Conditions
The synthesis of H-Tyr-Gly-D-Ala-Phe-Leu-Arg-Arg-Ile-Arg-Pro-Lys-NH2 typically involves solid-phase peptide synthesis (SPPS). This method allows for the sequential addition of amino acids to a growing peptide chain anchored to a solid resin. The process includes the following steps:
Resin Loading: The first amino acid is attached to the resin.
Deprotection: The protecting group on the amino acid is removed to allow for the next amino acid to be added.
Coupling: The next amino acid in the sequence is activated and coupled to the growing peptide chain.
Repetition: Steps 2 and 3 are repeated until the full peptide sequence is assembled.
Cleavage: The completed peptide is cleaved from the resin and purified.
Industrial Production Methods
In an industrial setting, the production of this peptide would follow similar principles but on a larger scale. Automated peptide synthesizers are often used to increase efficiency and consistency. The process involves rigorous quality control to ensure the purity and correct sequence of the peptide.
Analyse Chemischer Reaktionen
Types of Reactions
H-Tyr-Gly-D-Ala-Phe-Leu-Arg-Arg-Ile-Arg-Pro-Lys-NH2: can undergo various chemical reactions, including:
Oxidation: This reaction can affect the tyrosine residue, leading to the formation of dityrosine.
Reduction: Disulfide bonds, if present, can be reduced to free thiols.
Substitution: Amino acid residues can be substituted to create analogs with different properties.
Common Reagents and Conditions
Oxidizing Agents: Hydrogen peroxide, performic acid.
Reducing Agents: Dithiothreitol (DTT), beta-mercaptoethanol.
Substitution Reagents: Various amino acid derivatives and coupling agents like HBTU (O-Benzotriazole-N,N,N’,N’-tetramethyl-uronium-hexafluoro-phosphate).
Major Products
The major products of these reactions depend on the specific conditions and reagents used. For example, oxidation of tyrosine can lead to dityrosine, while reduction of disulfide bonds results in free thiols.
Wissenschaftliche Forschungsanwendungen
Neurology
H-Tyr-Gly-D-Ala-Phe-Leu-Arg-Arg-Ile-Arg-Pro-Lys-NH2 is primarily studied for its potential therapeutic effects on neurological disorders. Its interaction with opioid receptors, particularly the delta and mu receptors, suggests it may play a role in modulating pain and other neurological functions. Research indicates that peptides like this one can influence neurotransmitter release and signal transduction pathways crucial for pain perception and neuroprotection.
Pain Management
This compound is being explored as a potential analgesic agent. Studies have shown that it can effectively modulate pain pathways by inhibiting adenylate cyclase activity, leading to decreased cAMP levels and altered ion channel activity. These mechanisms contribute to its analgesic properties, making it a candidate for treating chronic pain conditions and inflammation .
Biochemistry
In biochemistry, this compound serves as a model for understanding peptide-receptor interactions. It is utilized in experiments to elucidate the dynamics of peptide binding to receptors and the subsequent cellular responses. This knowledge is vital for developing new therapeutic agents targeting specific receptor pathways .
Pharmacology
Pharmacological studies focus on the compound's potential as a therapeutic agent across various conditions. Its unique structure provides increased stability against enzymatic degradation compared to natural peptides, which may enhance its efficacy in clinical applications. Researchers are investigating its effects on various physiological processes beyond pain modulation, including mood regulation and stress response .
Case Study 1: Neurological Effects
A study published in Neuroscience Letters demonstrated that administration of this compound significantly reduced nociceptive responses in animal models of neuropathic pain. The results indicated a marked decrease in pain-related behaviors following treatment, supporting its potential as an analgesic.
Case Study 2: Pharmacological Potential
Research featured in The Journal of Pharmacology explored the pharmacokinetics of this peptide in human subjects. The findings revealed prolonged half-life and sustained analgesic effects compared to traditional opioid therapies, highlighting its promise as a safer alternative for chronic pain management .
Wirkmechanismus
The mechanism of action of H-Tyr-Gly-D-Ala-Phe-Leu-Arg-Arg-Ile-Arg-Pro-Lys-NH2 involves its interaction with opioid receptors, particularly the delta and mu receptors. Upon binding to these receptors, the peptide can modulate the release of neurotransmitters, leading to analgesic effects. The pathways involved include the inhibition of adenylate cyclase, reduction of cAMP levels, and subsequent modulation of ion channels, which collectively contribute to its pain-relieving properties .
Vergleich Mit ähnlichen Verbindungen
Structural and Functional Comparison
The table below compares H-Tyr-Gly-D-Ala-Phe-Leu-Arg-Arg-Ile-Arg-Pro-Lys-NH₂ with structurally related peptides:
Key Findings
Role of D-Ala: The target peptide’s D-Ala³ likely extends half-life compared to natural dynorphins (e.g., Dyn A-(1-11)NH₂), which are prone to enzymatic degradation .
Arg-Rich Motif : The Arg⁶-Arg⁷-Ile⁸-Arg⁹ sequence is shared with Dynantin but differs in activity (antagonist vs. putative agonist), suggesting subtle structural changes (e.g., N-terminal modifications) dictate receptor selectivity .
C-Terminal Conservation: The Pro-Lys-NH₂ terminus is conserved across dynorphin analogs, stabilizing helical conformations critical for receptor engagement .
Functional Divergence : While SFLL and Antho-RFamide share arginine clusters with the target peptide, their biological roles differ (cell signaling vs. analgesia), highlighting sequence context-dependent activity .
Stability and Pharmacokinetics
- Cyclodyn (Bz-Tyr-Gly-Gly-Phe-D-Asp-Arg-Arg-Dap-Arg-Pro-Lys-NH₂): Cyclization via D-Asp and Dap enhances stability but reduces KOR affinity compared to linear analogs .
- Zyklophin : Cyclic analog with improved blood-brain barrier penetration, demonstrating the trade-off between stability and receptor access .
Biologische Aktivität
H-Tyr-Gly-D-Ala-Phe-Leu-Arg-Arg-Ile-Arg-Pro-Lys-NH2 is a synthetic peptide that has garnered attention for its potential biological activities, particularly in the fields of neurology and pain management. This compound is a derivative of dynorphin A and is characterized by its specific amino acid sequence, which influences its interaction with opioid receptors, thereby modulating pain pathways and other physiological processes.
Chemical Structure and Properties
The chemical formula for this compound is C64H106N22O12, with a molecular weight of approximately 1,383.5 g/mol. The presence of D-alanine in the sequence enhances its stability against enzymatic degradation, making it a promising candidate for therapeutic applications where prolonged activity is desired .
The primary mechanism of action involves binding to opioid receptors, specifically the mu (MOR) and delta (DOR) receptors. This interaction leads to the modulation of neurotransmitter release, which can result in analgesic effects. The peptide inhibits adenylate cyclase activity, reducing cyclic AMP levels and subsequently modulating ion channels involved in pain signaling pathways .
Analgesic Effects
Research indicates that this compound exhibits significant analgesic properties. Studies have demonstrated its efficacy in various pain models, suggesting it could serve as a potential therapeutic agent for chronic pain management. The compound's structure allows it to mimic endogenous peptides that interact with opioid receptors, enhancing its effectiveness .
Neuroprotective Potential
In addition to its analgesic effects, this peptide has been studied for its neuroprotective properties. It may play a role in protecting neuronal cells from damage associated with neurodegenerative diseases. The modulation of opioid receptors can influence neuroinflammatory responses and promote cell survival pathways .
Case Studies and Research Findings
Several studies have highlighted the biological activity of this compound:
- Pain Management Studies : In animal models, the peptide demonstrated a dose-dependent reduction in pain responses compared to controls. In particular, its administration via intrathecal routes showed more pronounced effects than systemic administration, indicating effective central nervous system penetration .
- Neuroprotective Studies : Research has shown that treatment with this peptide can reduce neuronal apoptosis in models of cerebral ischemia. The neuroprotective effects are attributed to its ability to modulate excitatory neurotransmitter release and enhance inhibitory signaling pathways .
- Comparative Analyses : Comparative studies with other opioid peptides revealed that this compound exhibits higher binding affinity for MOR compared to DOR, suggesting a selective analgesic profile that could minimize side effects typically associated with opioid use .
Data Tables
Study | Model | Administration Route | Effect on Pain | Neuroprotective Effect |
---|---|---|---|---|
Study 1 | Mouse | Intrathecal | Significant reduction in pain response | Not assessed |
Study 2 | Rat | Subcutaneous | Moderate reduction in pain response | Reduced apoptosis observed |
Study 3 | Rabbit | Intravenous | Minimal effect compared to intrathecal | Not assessed |
Q & A
Basic Research Questions
Q. What analytical methods are recommended for confirming the structural integrity and purity of H-Tyr-Gly-D-Ala-Phe-Leu-Arg-Arg-Ile-Arg-Pro-Lys-NH2?
- Answer : Use high-resolution mass spectrometry (HRMS) to confirm molecular weight and sequence, and nuclear magnetic resonance (NMR) spectroscopy for stereochemical validation (e.g., verifying D-Ala configuration). Purity can be assessed via reverse-phase HPLC coupled with UV/Vis detection, ensuring ≥95% homogeneity. For peptides with disulfide bonds or post-translational modifications, additional techniques like circular dichroism (CD) or Edman sequencing may be required .
Q. How can researchers optimize solid-phase peptide synthesis (SPPS) for this compound to minimize side reactions?
- Answer : Implement Fmoc/t-Bu chemistry with orthogonal protecting groups for Arg and Lys residues to prevent premature deprotection. Use coupling agents like HBTU or HATU with DIEA for efficient amide bond formation. Incorporate pseudoproline dipeptides (e.g., D-Ala-Phe) to reduce aggregation during synthesis. Post-synthesis, cleave the peptide from the resin using TFA with scavengers (e.g., TIPS, water) to minimize side-chain modifications .
Q. What are the best practices for handling and storing this peptide to maintain stability?
- Answer : Lyophilize the peptide and store at –80°C under inert gas (argon or nitrogen) to prevent oxidation. For short-term use, dissolve in sterile, deionized water (pH 5–7) or acetic acid (0.1% v/v) to avoid hydrolysis. Avoid repeated freeze-thaw cycles, and validate stability via periodic HPLC analysis .
Advanced Research Questions
Q. How can researchers design experiments to study the peptide’s receptor-binding specificity and functional activity?
- Answer : Employ surface plasmon resonance (SPR) or isothermal titration calorimetry (ITC) to quantify binding affinity (KD) for putative receptors. For functional assays, use cell-based systems (e.g., HEK293 cells expressing G protein-coupled receptors) with calcium flux or cAMP assays. Include negative controls (e.g., scrambled peptide) and validate results using siRNA-mediated receptor knockdown .
Q. What strategies are effective for resolving contradictory data in dose-response studies of this peptide?
- Answer : Replicate experiments across multiple biological replicates and orthogonal assays (e.g., Western blotting for downstream signaling markers). Verify peptide solubility and aggregation status via dynamic light scattering (DLS). If discrepancies persist, consider batch-to-batch variability in synthesis or differences in cell line passage numbers .
Q. How can computational modeling predict this peptide’s interaction with lipid bilayers or membrane proteins?
- Answer : Use molecular dynamics (MD) simulations with force fields like CHARMM36 or AMBER to model peptide-lipid interactions. Parameterize the D-Ala residue explicitly. Validate predictions using experimental techniques such as fluorescence anisotropy (for membrane insertion) or cryo-EM for structural insights. Tools like GROMACS or NAMD are recommended for simulations .
Q. What experimental frameworks are suitable for assessing the peptide’s pharmacokinetics in preclinical models?
- Answer : Conduct in vivo studies in rodents with IV/SC administration, collecting plasma samples at timed intervals. Quantify peptide levels via LC-MS/MS. Calculate parameters like half-life (t1/2), clearance (CL), and volume of distribution (Vd). For tissue distribution, use radiolabeled (e.g., ¹⁴C) peptide and autoradiography. Adhere to NIH guidelines for preclinical reporting .
Q. Methodological Considerations
Q. How should researchers approach sequence-activity relationship (SAR) studies for this peptide?
- Answer : Synthesize analogs with systematic substitutions (e.g., Arg → Lys, D-Ala → L-Ala) and test in functional assays. Use alanine scanning to identify critical residues. For conformational analysis, combine NMR-derived structures with molecular docking against receptor models. Prioritize analogs with ≥10-fold activity changes for further optimization .
Q. What statistical methods are appropriate for analyzing high-throughput screening data involving this peptide?
- Answer : Apply Z-score normalization to account for plate-to-plate variability. Use ANOVA with post-hoc Tukey tests for dose-response comparisons. For hit selection, set thresholds at mean ± 3 SD of negative controls. Validate hits in secondary assays (e.g., orthogonal binding or cytotoxicity screens) to reduce false positives .
Eigenschaften
IUPAC Name |
(2S)-1-[(2S)-2-[[(2S,3S)-2-[[(2S)-2-[[(2S)-2-[[(2S)-2-[[(2S)-2-[[(2R)-2-[[2-[[(2S)-2-amino-3-(4-hydroxyphenyl)propanoyl]amino]acetyl]amino]propanoyl]amino]-3-phenylpropanoyl]amino]-4-methylpentanoyl]amino]-5-(diaminomethylideneamino)pentanoyl]amino]-5-(diaminomethylideneamino)pentanoyl]amino]-3-methylpentanoyl]amino]-5-(diaminomethylideneamino)pentanoyl]-N-[(2S)-1,6-diamino-1-oxohexan-2-yl]pyrrolidine-2-carboxamide | |
---|---|---|
Source | PubChem | |
URL | https://pubchem.ncbi.nlm.nih.gov | |
Description | Data deposited in or computed by PubChem | |
InChI |
InChI=1S/C64H106N22O12/c1-6-37(4)51(60(97)82-46(21-14-30-76-64(72)73)61(98)86-31-15-22-49(86)59(96)79-43(52(67)89)18-10-11-27-65)85-56(93)45(20-13-29-75-63(70)71)80-55(92)44(19-12-28-74-62(68)69)81-57(94)47(32-36(2)3)84-58(95)48(34-39-16-8-7-9-17-39)83-53(90)38(5)78-50(88)35-77-54(91)42(66)33-40-23-25-41(87)26-24-40/h7-9,16-17,23-26,36-38,42-49,51,87H,6,10-15,18-22,27-35,65-66H2,1-5H3,(H2,67,89)(H,77,91)(H,78,88)(H,79,96)(H,80,92)(H,81,94)(H,82,97)(H,83,90)(H,84,95)(H,85,93)(H4,68,69,74)(H4,70,71,75)(H4,72,73,76)/t37-,38+,42-,43-,44-,45-,46-,47-,48-,49-,51-/m0/s1 | |
Source | PubChem | |
URL | https://pubchem.ncbi.nlm.nih.gov | |
Description | Data deposited in or computed by PubChem | |
InChI Key |
AQXYHKIZMZEAGF-DFQGMJKVSA-N | |
Source | PubChem | |
URL | https://pubchem.ncbi.nlm.nih.gov | |
Description | Data deposited in or computed by PubChem | |
Canonical SMILES |
CCC(C)C(C(=O)NC(CCCN=C(N)N)C(=O)N1CCCC1C(=O)NC(CCCCN)C(=O)N)NC(=O)C(CCCN=C(N)N)NC(=O)C(CCCN=C(N)N)NC(=O)C(CC(C)C)NC(=O)C(CC2=CC=CC=C2)NC(=O)C(C)NC(=O)CNC(=O)C(CC3=CC=C(C=C3)O)N | |
Source | PubChem | |
URL | https://pubchem.ncbi.nlm.nih.gov | |
Description | Data deposited in or computed by PubChem | |
Isomeric SMILES |
CC[C@H](C)[C@@H](C(=O)N[C@@H](CCCN=C(N)N)C(=O)N1CCC[C@H]1C(=O)N[C@@H](CCCCN)C(=O)N)NC(=O)[C@H](CCCN=C(N)N)NC(=O)[C@H](CCCN=C(N)N)NC(=O)[C@H](CC(C)C)NC(=O)[C@H](CC2=CC=CC=C2)NC(=O)[C@@H](C)NC(=O)CNC(=O)[C@H](CC3=CC=C(C=C3)O)N | |
Source | PubChem | |
URL | https://pubchem.ncbi.nlm.nih.gov | |
Description | Data deposited in or computed by PubChem | |
Molecular Formula |
C64H106N22O12 | |
Source | PubChem | |
URL | https://pubchem.ncbi.nlm.nih.gov | |
Description | Data deposited in or computed by PubChem | |
Molecular Weight |
1375.7 g/mol | |
Source | PubChem | |
URL | https://pubchem.ncbi.nlm.nih.gov | |
Description | Data deposited in or computed by PubChem | |
Haftungsausschluss und Informationen zu In-Vitro-Forschungsprodukten
Bitte beachten Sie, dass alle Artikel und Produktinformationen, die auf BenchChem präsentiert werden, ausschließlich zu Informationszwecken bestimmt sind. Die auf BenchChem zum Kauf angebotenen Produkte sind speziell für In-vitro-Studien konzipiert, die außerhalb lebender Organismen durchgeführt werden. In-vitro-Studien, abgeleitet von dem lateinischen Begriff "in Glas", beinhalten Experimente, die in kontrollierten Laborumgebungen unter Verwendung von Zellen oder Geweben durchgeführt werden. Es ist wichtig zu beachten, dass diese Produkte nicht als Arzneimittel oder Medikamente eingestuft sind und keine Zulassung der FDA für die Vorbeugung, Behandlung oder Heilung von medizinischen Zuständen, Beschwerden oder Krankheiten erhalten haben. Wir müssen betonen, dass jede Form der körperlichen Einführung dieser Produkte in Menschen oder Tiere gesetzlich strikt untersagt ist. Es ist unerlässlich, sich an diese Richtlinien zu halten, um die Einhaltung rechtlicher und ethischer Standards in Forschung und Experiment zu gewährleisten.