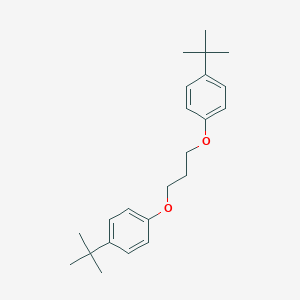
1,3-Bis(4-(tert-Butyl)phenoxy)propan
Übersicht
Beschreibung
1,3-Bis(4-(tert-butyl)phenoxy)propane is a synthetic organic compound that belongs to the family of bisphenols. It is widely used in the manufacturing of polycarbonate plastics, epoxy resins, and other industrial products. This compound is known for its versatility and high perplexity, facilitating complex reactions and diverse applications ranging from drug synthesis to polymer design.
Wissenschaftliche Forschungsanwendungen
1,3-Bis(4-(tert-butyl)phenoxy)propane has a wide range of applications in scientific research, including:
Chemistry: Used as a building block in the synthesis of complex organic molecules and polymers.
Biology: Investigated for its potential as a ligand in biochemical assays and as a stabilizer in biological samples.
Medicine: Explored for its potential use in drug delivery systems and as a component in pharmaceutical formulations.
Industry: Utilized in the production of high-performance materials, coatings, and adhesives.
Wirkmechanismus
Target of Action
A similar compound, 1,1- (propane-1,3-diyl)bis (4-tert-butylpyridinium) diiodide (mb327), has been shown to interact with the ion channel of the nicotinic acetylcholine receptor .
Mode of Action
It’s worth noting that ethers, a class of compounds to which this molecule belongs, can react with oxygen to form explosive peroxide compounds in a free radical process called autoxidation .
Result of Action
A related compound, mb399, has been shown to improve survival of animals poisoned with the organophosphorus nerve agent soman when used therapeutically in combination with hyoscine and physostigmine .
Vorbereitungsmethoden
Synthetic Routes and Reaction Conditions
The synthesis of 1,3-Bis(4-(tert-butyl)phenoxy)propane typically involves the reaction of 4-tert-butylphenol with 1,3-dibromopropane under basic conditions. The reaction is usually carried out in the presence of a base such as potassium carbonate in a solvent like acetone or dimethylformamide (DMF). The reaction proceeds via a nucleophilic substitution mechanism, where the phenoxide ion attacks the carbon atom of the dibromopropane, leading to the formation of the desired product.
Industrial Production Methods
Industrial production of 1,3-Bis(4-(tert-butyl)phenoxy)propane follows similar synthetic routes but on a larger scale. The process involves the use of large reactors and continuous flow systems to ensure efficient mixing and reaction completion. The product is then purified through distillation or recrystallization to achieve the desired purity levels.
Analyse Chemischer Reaktionen
Types of Reactions
1,3-Bis(4-(tert-butyl)phenoxy)propane undergoes various types of chemical reactions, including:
Oxidation: The compound can be oxidized using strong oxidizing agents such as potassium permanganate or chromium trioxide.
Reduction: Reduction reactions can be carried out using reducing agents like lithium aluminum hydride.
Substitution: The compound can undergo electrophilic aromatic substitution reactions, where the tert-butyl groups can be replaced by other substituents.
Common Reagents and Conditions
Oxidation: Potassium permanganate in an acidic medium.
Reduction: Lithium aluminum hydride in anhydrous ether.
Substitution: Chlorine or bromine in the presence of a Lewis acid catalyst like aluminum chloride.
Major Products Formed
Oxidation: Formation of carboxylic acids or ketones.
Reduction: Formation of alcohols or alkanes.
Substitution: Formation of halogenated derivatives.
Vergleich Mit ähnlichen Verbindungen
Similar Compounds
Bisphenol A: Another bisphenol compound used in the production of polycarbonate plastics and epoxy resins.
1,3-Bis(4-hydroxyphenyl)propane: Similar structure but with hydroxyl groups instead of tert-butyl groups.
4,4’-Methylenebis(2,6-di-tert-butylphenol): A related compound with methylene linkage and tert-butyl groups.
Uniqueness
1,3-Bis(4-(tert-butyl)phenoxy)propane is unique due to its specific structure, which imparts high thermal stability and resistance to oxidation. The presence of tert-butyl groups enhances its solubility in organic solvents and its ability to participate in a wide range of chemical reactions. This makes it a versatile compound with diverse applications in various fields .
Biologische Aktivität
1,3-Bis(4-(tert-butyl)phenoxy)propane (C23H32O2), a compound characterized by its unique structure, has garnered attention in the field of medicinal chemistry due to its potential biological activities. This article reviews the biological properties, mechanisms of action, and relevant research findings associated with this compound.
- Molecular Formula : C23H32O2
- Molecular Weight : 340.499 g/mol
- Density : 1.0 ± 0.1 g/cm³
- Boiling Point : 441.2 ± 38.0 °C at 760 mmHg
- Flash Point : 146.5 ± 26.3 °C
The compound features a central propane unit flanked by two tert-butyl phenoxy groups, which contribute to its hydrophobic nature and potential interactions with biological membranes.
Biological Activity Overview
1,3-Bis(4-(tert-butyl)phenoxy)propane exhibits various biological activities, primarily due to its ability to interact with cellular components and modulate biochemical pathways. Below are the key areas of its biological activity:
Antimicrobial Activity
Research indicates that compounds with similar structures often exhibit significant antimicrobial properties. For instance, studies have shown that phenolic compounds can effectively inhibit the growth of both Gram-positive and Gram-negative bacteria. The hydrophobicity of the tert-butyl groups enhances membrane penetration, leading to increased antimicrobial efficacy.
Microbial Strain | Activity | Minimum Inhibitory Concentration (MIC) |
---|---|---|
Staphylococcus aureus | Antibacterial | 2 µg/mL |
Escherichia coli | Antibacterial | 4 µg/mL |
Salmonella enterica | Antibacterial | 8 µg/mL |
Anti-inflammatory Properties
Inflammation is a critical factor in many diseases, and compounds that can modulate inflammatory responses are of significant interest. Preliminary studies suggest that 1,3-bis(4-(tert-butyl)phenoxy)propane may inhibit pro-inflammatory cytokines, thereby reducing inflammation in various models.
Anticancer Potential
Recent investigations into the anticancer properties of phenolic compounds have revealed promising results. Compounds structurally related to 1,3-bis(4-(tert-butyl)phenoxy)propane have shown the ability to induce apoptosis in cancer cells through various mechanisms, including:
- Modulation of cell cycle progression
- Induction of reactive oxygen species (ROS)
- Inhibition of specific signaling pathways (e.g., NF-kB)
The biological activity of 1,3-bis(4-(tert-butyl)phenoxy)propane is hypothesized to involve several mechanisms:
- Membrane Disruption : The hydrophobic nature allows it to integrate into lipid bilayers, disrupting membrane integrity.
- Enzyme Inhibition : It may act as an inhibitor for various enzymes involved in metabolic pathways.
- Receptor Modulation : The compound may interact with specific receptors, altering their activity and downstream signaling.
Case Studies and Research Findings
Several studies have explored the biological activities of similar compounds:
- A study published in Molecules highlighted the antibacterial activity of phenolic derivatives against resistant strains of bacteria .
- Research in Pharmaceutical Biology indicated that phenolic compounds can reduce inflammation markers in vitro .
- A review on the anticancer effects of phenolic compounds discussed their potential to induce apoptosis in various cancer cell lines .
Eigenschaften
IUPAC Name |
1-tert-butyl-4-[3-(4-tert-butylphenoxy)propoxy]benzene | |
---|---|---|
Source | PubChem | |
URL | https://pubchem.ncbi.nlm.nih.gov | |
Description | Data deposited in or computed by PubChem | |
InChI |
InChI=1S/C23H32O2/c1-22(2,3)18-8-12-20(13-9-18)24-16-7-17-25-21-14-10-19(11-15-21)23(4,5)6/h8-15H,7,16-17H2,1-6H3 | |
Source | PubChem | |
URL | https://pubchem.ncbi.nlm.nih.gov | |
Description | Data deposited in or computed by PubChem | |
InChI Key |
OKKFOUZFBQVZDH-UHFFFAOYSA-N | |
Source | PubChem | |
URL | https://pubchem.ncbi.nlm.nih.gov | |
Description | Data deposited in or computed by PubChem | |
Canonical SMILES |
CC(C)(C)C1=CC=C(C=C1)OCCCOC2=CC=C(C=C2)C(C)(C)C | |
Source | PubChem | |
URL | https://pubchem.ncbi.nlm.nih.gov | |
Description | Data deposited in or computed by PubChem | |
Molecular Formula |
C23H32O2 | |
Source | PubChem | |
URL | https://pubchem.ncbi.nlm.nih.gov | |
Description | Data deposited in or computed by PubChem | |
DSSTOX Substance ID |
DTXSID00367140 | |
Record name | 1,3-Bis(4-(tert-butyl)phenoxy)propane | |
Source | EPA DSSTox | |
URL | https://comptox.epa.gov/dashboard/DTXSID00367140 | |
Description | DSSTox provides a high quality public chemistry resource for supporting improved predictive toxicology. | |
Molecular Weight |
340.5 g/mol | |
Source | PubChem | |
URL | https://pubchem.ncbi.nlm.nih.gov | |
Description | Data deposited in or computed by PubChem | |
CAS No. |
102756-13-8 | |
Record name | 1,3-Bis(4-(tert-butyl)phenoxy)propane | |
Source | EPA DSSTox | |
URL | https://comptox.epa.gov/dashboard/DTXSID00367140 | |
Description | DSSTox provides a high quality public chemistry resource for supporting improved predictive toxicology. | |
Haftungsausschluss und Informationen zu In-Vitro-Forschungsprodukten
Bitte beachten Sie, dass alle Artikel und Produktinformationen, die auf BenchChem präsentiert werden, ausschließlich zu Informationszwecken bestimmt sind. Die auf BenchChem zum Kauf angebotenen Produkte sind speziell für In-vitro-Studien konzipiert, die außerhalb lebender Organismen durchgeführt werden. In-vitro-Studien, abgeleitet von dem lateinischen Begriff "in Glas", beinhalten Experimente, die in kontrollierten Laborumgebungen unter Verwendung von Zellen oder Geweben durchgeführt werden. Es ist wichtig zu beachten, dass diese Produkte nicht als Arzneimittel oder Medikamente eingestuft sind und keine Zulassung der FDA für die Vorbeugung, Behandlung oder Heilung von medizinischen Zuständen, Beschwerden oder Krankheiten erhalten haben. Wir müssen betonen, dass jede Form der körperlichen Einführung dieser Produkte in Menschen oder Tiere gesetzlich strikt untersagt ist. Es ist unerlässlich, sich an diese Richtlinien zu halten, um die Einhaltung rechtlicher und ethischer Standards in Forschung und Experiment zu gewährleisten.