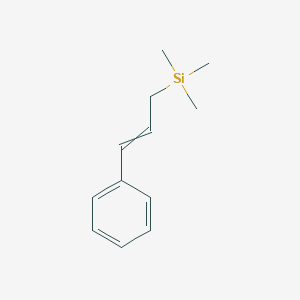
Cinnamyltrimethylsilan
Übersicht
Beschreibung
Wissenschaftliche Forschungsanwendungen
Cinnamyltrimethylsilane has found applications in various scientific research fields:
Chemistry: It is used as a reagent in organic synthesis, particularly in the formation of carbon-silicon bonds.
Biology: The compound is explored for its potential in modifying biological molecules and studying their interactions.
Medicine: Research is ongoing to investigate its potential as a precursor for drug development and delivery systems.
Industry: Cinnamyltrimethylsilane is utilized in the production of specialty chemicals and materials, including polymers and coatings
Vorbereitungsmethoden
Synthetic Routes and Reaction Conditions: Cinnamyltrimethylsilane can be synthesized through several methods. One common approach involves the reaction of cinnamyl chloride with trimethylchlorosilane in the presence of a base such as magnesium in tetrahydrofuran. The reaction is typically carried out at low temperatures, around -78°C to 25°C, and yields the desired product with high efficiency .
Industrial Production Methods: Industrial production of cinnamyltrimethylsilane often involves similar synthetic routes but on a larger scale. The use of continuous flow reactors and optimized reaction conditions can enhance the yield and purity of the product. Additionally, the reaction of benzyl chloride with trimethylsilyl chloride is another method employed in industrial settings .
Analyse Chemischer Reaktionen
Types of Reactions: Cinnamyltrimethylsilane undergoes various chemical reactions, including:
Oxidation: The compound can be oxidized to form corresponding silanols or siloxanes.
Reduction: Reduction reactions can convert cinnamyltrimethylsilane to its corresponding hydrosilane derivatives.
Substitution: The compound can participate in substitution reactions, where the trimethylsilyl group is replaced by other functional groups.
Common Reagents and Conditions:
Oxidation: Reagents such as hydrogen peroxide or peracids are commonly used under mild conditions.
Reduction: Reducing agents like lithium aluminum hydride or sodium borohydride are employed.
Substitution: Halogenating agents or nucleophiles are used in substitution reactions.
Major Products Formed: The major products formed from these reactions include silanols, siloxanes, hydrosilanes, and various substituted derivatives depending on the reaction conditions and reagents used .
Wirkmechanismus
The mechanism of action of cinnamyltrimethylsilane involves its ability to participate in various chemical reactions due to the presence of the reactive trimethylsilyl group. This group can undergo nucleophilic substitution, oxidation, and reduction reactions, making the compound versatile in different chemical environments. The molecular targets and pathways involved depend on the specific application and reaction conditions .
Vergleich Mit ähnlichen Verbindungen
Trimethylsilane: An organosilicon compound with the formula (CH3)3SiH, known for its reactivity and use in the semiconductor industry.
Triethylsilane: Similar to trimethylsilane but with ethyl groups, used as a reducing agent in organic synthesis.
Cinnamylsilane: A compound with a similar cinnamyl group but different silicon-containing moieties.
Uniqueness: Its ability to undergo a variety of chemical reactions and its use in diverse fields make it a valuable compound for research and industrial applications .
Eigenschaften
IUPAC Name |
trimethyl(3-phenylprop-2-enyl)silane | |
---|---|---|
Source | PubChem | |
URL | https://pubchem.ncbi.nlm.nih.gov | |
Description | Data deposited in or computed by PubChem | |
InChI |
InChI=1S/C12H18Si/c1-13(2,3)11-7-10-12-8-5-4-6-9-12/h4-10H,11H2,1-3H3 | |
Source | PubChem | |
URL | https://pubchem.ncbi.nlm.nih.gov | |
Description | Data deposited in or computed by PubChem | |
InChI Key |
QSOKYXQHVHZSER-UHFFFAOYSA-N | |
Source | PubChem | |
URL | https://pubchem.ncbi.nlm.nih.gov | |
Description | Data deposited in or computed by PubChem | |
Canonical SMILES |
C[Si](C)(C)CC=CC1=CC=CC=C1 | |
Source | PubChem | |
URL | https://pubchem.ncbi.nlm.nih.gov | |
Description | Data deposited in or computed by PubChem | |
Molecular Formula |
C12H18Si | |
Source | PubChem | |
URL | https://pubchem.ncbi.nlm.nih.gov | |
Description | Data deposited in or computed by PubChem | |
Molecular Weight |
190.36 g/mol | |
Source | PubChem | |
URL | https://pubchem.ncbi.nlm.nih.gov | |
Description | Data deposited in or computed by PubChem | |
Retrosynthesis Analysis
AI-Powered Synthesis Planning: Our tool employs the Template_relevance Pistachio, Template_relevance Bkms_metabolic, Template_relevance Pistachio_ringbreaker, Template_relevance Reaxys, Template_relevance Reaxys_biocatalysis model, leveraging a vast database of chemical reactions to predict feasible synthetic routes.
One-Step Synthesis Focus: Specifically designed for one-step synthesis, it provides concise and direct routes for your target compounds, streamlining the synthesis process.
Accurate Predictions: Utilizing the extensive PISTACHIO, BKMS_METABOLIC, PISTACHIO_RINGBREAKER, REAXYS, REAXYS_BIOCATALYSIS database, our tool offers high-accuracy predictions, reflecting the latest in chemical research and data.
Strategy Settings
Precursor scoring | Relevance Heuristic |
---|---|
Min. plausibility | 0.01 |
Model | Template_relevance |
Template Set | Pistachio/Bkms_metabolic/Pistachio_ringbreaker/Reaxys/Reaxys_biocatalysis |
Top-N result to add to graph | 6 |
Feasible Synthetic Routes
Q1: What makes Cinnamyltrimethylsilane useful in synthesizing SERMs like Lasofoxifene and Nafoxidine?
A1: Cinnamyltrimethylsilane participates in a unique three-component coupling reaction that streamlines the synthesis of Lasofoxifene and Nafoxidine [, ]. This reaction, facilitated by Lewis acids like Hafnium(IV) chloride (HfCl₄), combines Cinnamyltrimethylsilane with an aromatic aldehyde (e.g., 4-pivaloyloxybenzaldehyde) and an aryl ether (e.g., anisole) in a single pot. This one-pot approach significantly reduces the number of synthetic steps, making the process more efficient and cost-effective compared to traditional methods.
Q2: Can you elaborate on the role of Cinnamyltrimethylsilane in this three-component coupling reaction?
A2: Cinnamyltrimethylsilane acts as a nucleophilic partner in the reaction. The Lewis acid activates the aldehyde, making it susceptible to attack by the Cinnamyltrimethylsilane. This forms an intermediate which subsequently reacts with the aryl ether, ultimately leading to the formation of a 3,4,4-triaryl-1-butene derivative [, ]. This compound then serves as a common intermediate for synthesizing various SERMs, including Lasofoxifene and Nafoxidine, through subsequent cyclization, elimination, and double-bond migration steps.
Q3: Beyond Lasofoxifene and Nafoxidine, are there other SERMs synthesized using Cinnamyltrimethylsilane?
A3: Yes, research indicates that the same three-component coupling strategy utilizing Cinnamyltrimethylsilane has been successfully employed in the synthesis of Droloxifene [] and Tamoxifen [], further highlighting the versatility of this approach in accessing diverse SERM structures.
Haftungsausschluss und Informationen zu In-Vitro-Forschungsprodukten
Bitte beachten Sie, dass alle Artikel und Produktinformationen, die auf BenchChem präsentiert werden, ausschließlich zu Informationszwecken bestimmt sind. Die auf BenchChem zum Kauf angebotenen Produkte sind speziell für In-vitro-Studien konzipiert, die außerhalb lebender Organismen durchgeführt werden. In-vitro-Studien, abgeleitet von dem lateinischen Begriff "in Glas", beinhalten Experimente, die in kontrollierten Laborumgebungen unter Verwendung von Zellen oder Geweben durchgeführt werden. Es ist wichtig zu beachten, dass diese Produkte nicht als Arzneimittel oder Medikamente eingestuft sind und keine Zulassung der FDA für die Vorbeugung, Behandlung oder Heilung von medizinischen Zuständen, Beschwerden oder Krankheiten erhalten haben. Wir müssen betonen, dass jede Form der körperlichen Einführung dieser Produkte in Menschen oder Tiere gesetzlich strikt untersagt ist. Es ist unerlässlich, sich an diese Richtlinien zu halten, um die Einhaltung rechtlicher und ethischer Standards in Forschung und Experiment zu gewährleisten.